B13
Chloroform
Hector D. Garcia, Ph.D.
Johnson Space Center Toxicology Group
Medical Operations Branch
Houston, Texas
PHYSICAL AND CHEMICAL PROPERTIES
Chloroform is a clear, colorless, volatile and mobile, highly refractive, dense liquid with a characteristic pleasant, nonirritating odor and a slight, sweet taste (ATSDR 1997).
Formula: |
CHCl3 |
CAS no.: |
67-66-3 |
Chemical name: |
Trichloromethane |
Synonyms: |
Chloroform, trichloroform, formyl trichloride, methenyl chloride, methenyl trichloride, methane trichloride, methyl trichloride, NCI-C02686, Freon 20, R-20, TCM |
Molecular weight: |
119.38 |
Boiling point: |
61.3°C |
Melting point: |
–63.2°C |
Liquid density: |
1.485 g/m3 |
Vapor density: |
4.36 (air = 1) |
Vapor pressure: |
159 torr at 20°C |
Solubility: |
1 mL dissolves in 200 mL at 25°C |
Odor threshold: |
85 ppm (vapor) Miscible with alcohol, benzene, ether, petroleum ether, carbon tetrachloride, carbon disulfide, oils. |
Conversion factors at 20°C, 1 atm: |
1 ppm = 4.96 mg/m3 |
|
1 mg/m3 = 0.20 ppm |
OCCURRENCE AND USE
Chloroform is used as a raw material in the chemical industry for the manufacture of such materials as fluorocarbons, resins, and plastics; as an extractant for fats, oils, greases, resins, lacquers, rubber, alkaloids, gums, waxes, guttapercha, penicillin, vitamins, flavors, floor polishes, and adhesives; as a pharmaceutical solvent and dry cleaning spot remover; and as an intermediate in the manufacture of dyes and pesticides (ATSDR 1997). In the past, chloroform was used as a general anesthetic, a fire extinguisher, and a flavoring agent in toothpastes and cough syrups (ACGIH 1991). Trace amounts of chloroform are present in drinking water and in wastewater from sewage-treatment plants as a by-product of chlorine treatment to kill bacteria. Trace amounts of chloroform are also found almost ubiquitously in the environment. Small amounts of chloroform are sometimes carried on board the space shuttle as part of mid-deck or module experiments. Chloroform has been detected in the shuttle atmosphere in 6 of 27 missions at concentrations of 0.002 to 0.03 mg/m3 (Huntoon 1987, 1993) and, in more recent missions, in about 10% of air samples at concentrations of 0.01 to 0.1 mg/m3 (James et al. 1994).
TOXICOKINETICS AND METABOLISM
Considerable data are available on the uptake, processing, and elimination of chloroform in several species. The weight of evidence indicates that chloroform is rapidly distributed throughout the body and that its toxic effects are more dependent on the dose rate than the total dose or the route of administration.
Absorption
Due to chloroform's relatively high vapor pressure and high blood/air partition coefficient (8 to 10.3 at 37°C), inhalation is a primary route of entry into the body (EPA 1985). Raabe (1988) measured the uptake of ambient concentrations of chloroform (labeled with 14C) in air inhaled through the nose (45.6%) and the mouth (49.6%) by four human subjects. Chloroform is also rapidly absorbed through the gastrointestinal tract from foodstuffs and drinking water (EPA 1985). Absorption of orally administered chloroform might be affected by the vehicle in which it is dissolved. In mice, tissue concentrations of chloroform after gavage dosing were consistently greater for aqueous versus corn-oil vehicles (Dix et al. 1997). Absorption of chloroform through the skin is significant (329 µmoles/min/cm2 of skin exposed to the liquid) (EPA 1985).
Distribution
In humans (Smith et al. 1973) and animals (Cohen 1971; Brown et al. 1974), chloroform absorbed either by inhalation or orally is distributed to all tissues with relative tissue concentrations of body fat> brain> liver> kidney> blood, as expected owing to the lipophilic nature of chloroform. In studies in mice, the relative distribution in the organs was dependent on route of administration; oral dosing resulted in the highest concentrations being in the liver, possibly due to a first-pass effect, the time between dosing and measurement, and the metabolism and covalent binding of metabolites to cellular macromolecules (Brown et al. 1974; Taylor et al. 1974).
Excretion
Chloroform was detected in the exhaled air of volunteers exposed to a normal environment, to heavy automobile traffic, or to 2 h in a dry-cleaning establishment (Gordon et al. 1988). High chloroform concentrations in the breath corresponded to high exposure concentrations. The calculated biological half-time for chloroform was 7.9 h.
Excretion of radioactivity in mice and rats was monitored for 48 h following exposure to 14C-labeled chloroform at 10, 89, and 366 ppm (mice) or 93, 356, and 1041 ppm (rats) (Corley et al. 1990). In this study, 92% to 99% of the total radioactivity was recovered in mice, and 58% to 98% was recovered in rats; percentage recovery decreased with increasing exposure. Of the total radioactivity, the percentages recovered as exhaled 14C-labeled carbon dioxide were 80% to 85% for mice and 48% to 85% for rats. The fractions recovered as 14C-labeled chloroform were 0.4% to 8% for mice and 2% to 42% for rats. The fractions recovered as urinary and fecal metabolites were 8% to 11% and 0.6% to 1.4% for mice and 6.4% to 8.9% and 0.6% to 1.1% for rats, respectively. A 4-fold increase in exposure concentration was followed by a 50- and 20-fold increase in the amount of exhaled, unmetabolized chloroform in mice and rats, respectively.
Metabolism
The metabolism of chloroform has been extensively studied and is fairly well understood. In humans, approximately 50% of an oral dose of 0.5 g chloroform was metabolized to carbon dioxide (Fry et al. 1972). Metabolism was dose
dependent, decreasing with higher exposure. A first-pass effect was observed after oral exposure (Chiou 1975). Approximately 38% of the dose was converted in the liver, and ≤ 17% was exhaled unchanged from the lungs. In a physiologically based pharmacokinetic modeling study of chloroform, Corley et al. (1990) defined in vivo metabolic rate constants (Vmax C = 15.7 mg/kg/h, Km = 0.448 mg) for humans by using experimental results obtained in rats and mice exposed to chloroform by inhalation and enzymatic studies in human tissues in vitro. Their results predicted that metabolic activation of chloroform to its toxic intermediate, phosgene, was slower in humans than in rodents (ATSDR 1997).
Chloroform can be metabolized aerobically and anaerobically as shown below:
Major Pathway (Aerobic).Source: Adapted from ATSDR (1997)
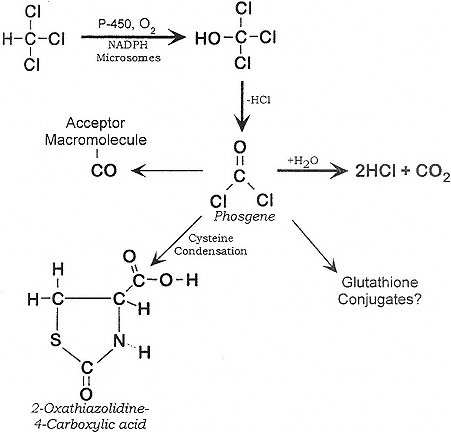
Minor Pathway (Anaerobic). Source: Adapted from ATSDR (1997)
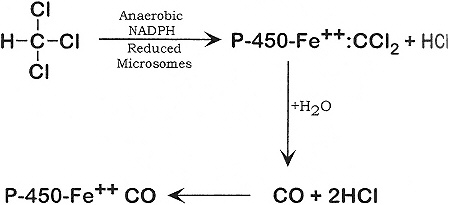
The production of CO2 by the aerobic pathway accounts for up to 85% of administered chloroform in mice, 65% in rats, and lesser amounts (28%) in squirrel monkeys and humans (50%) (Brown et al. 1974; Taylor et al. 1974). In mice, Brown et al. found greater amounts of radiolabeled chloroform in the kidneys of males than of females. Similarly, Culliford and Hewitt (1957) found that chloroform accumulated and metabolized to a greater extent in the renal cortex of males than of females; the results might have been influenced by testosterone concentrations. This effect was not observed in any other species. These species and sex differences in metabolism, distribution, and binding point out the dangers and difficulties in extrapolating studies in lower animals to humans.
Metabolism studies by Pohl (1977) and Stevens and Anders (1981) indicated that chloroform was exhaled from the lungs or was converted to phosgene in the liver and kidneys by cytochrome P-450 (Branchflower et al. 1984; Smith and Hook 1984). Phosgene might react with cellular elements, including lipids and proteins of the endoplasmic reticulum proximate to the cytochrome P-450. In phenobarbital-pretreated Sprague-Dawley rats, chloroform exposure yielded a covalent adduct to a single phospholipid, identified as phosphatidylethanolamine, in liver mitochondria (Guastedisegni et al. 1998). It was further demonstrated that chloroform can induce lipid peroxidation and inactivation of cytochrome P-450 in rat-liver microsomes under aerobic conditions (DeGroot and Noll 1989). This mechanism might also contribute to chloroform-induced hepatotoxicity in rats, although phosgene and other active metabolites are primarily responsible. The conversion of chloroform to reactive metabolites
occurs in nuclear preparations, as well as in microsomes (Gomez and Castro 1980). Covalent binding of chloroform to lipids can occur under anaerobic and aerobic conditions; binding to protein occurs only under aerobic conditions (Testai et al. 1987).
Covalent binding of chloroform metabolites to microsomal protein in vitro was intensified by microsomal enzyme inducers and prevented by glutathione (Brown et al. 1974). It was proposed that the reaction of chloroform metabolites with glutathione might act as a detoxifying mechanism. Phosgene might combine with two molecules of reduced glutathione (GSH) to form diglutathionyl dithiocarbonate, which is further metabolized in the kidneys (Sipes et al. 1977; Wolf et al. 1977). Chloroform doses that caused liver glutathione depletion produced liver necrosis (Docks and Krishna 1976). Furthermore, chloroform has been found to be more hepatotoxic in fasted animals, possibly due to decreased glutathione content in the liver (Brown et al. 1974; Docks and Krishna 1976; Wang et al. 1995). That might explain the clinical finding of severe acute hepatotoxicity in women exposed to chloroform via anesthesia during prolonged parturition.
Evidence that chloroform is metabolized at its carbon-hydrogen bond is provided by experiments using the deuterated derivative of chloroform (McCarty et al. 1979; Pohl et al. 1980; Branchflower et al. 1984). Deuterated chloroform was one-half to one-third as cytotoxic as chloroform, and its conversion to phosgene was much slower. The results confirmed that the toxicity of chloroform is primarily due to its metabolites (ATSDR 1997).
A recent in vitro study of mice hepatic microsomes indicated that a reductive pathway might play an important role in chloroform hepatotoxicity (Testai et al. 1990). It was demonstrated that radical chloroform metabolites bind to macromolecules (proteins, lipids) and the process can be inhibited by reduced glutathione (ATSDR 1997).
The final product of the aerobic metabolic pathway of chloroform is carbon dioxide (Fry et al. 1972; Brown et al. 1974), which is mostly eliminated through the lung, but some is incorporated into endogenous metabolites and excreted as bicarbonate, urea, methionine, and other amino acids (Brown et al. 1974). Inorganic chloride ion is an end product of chloroform metabolism found in the urine (Van Dyke et al. 1964). Carbon monoxide was a minor product of the anaerobic metabolism of chloroform in vitro (Ahmed et al. 1977) and in vivo in rats (Anders et al. 1978; ATSDR 1997).
Interspecies differences in the rate of chloroform conversion were observed in mice, rats, and squirrel monkeys. The conversion of chloroform to carbon dioxide was highest in mice (85%) and lowest in squirrel monkeys (28%) (Brown et al. 1974). Similarly, because of the lower relative rates of chloro-
form metabolism, ventilation, and cardiac output (per kilogram of body weight) in the larger species, physiologically based pharmacokinetic (PBPK) calculations indicated that exposure to equivalent concentrations of chloroform vapor would lead to a lower delivered dose of active metabolites in humans compared with rats, which would have a lower delivered dose than mice (Corley et al. 1990; ATSDR 1997).
TOXICITY SUMMARY
Chloroform has pronounced effects on the central nervous system (CNS), most of which are reversible upon cessation of exposure. Short-term exposure to high concentrations causes liver necrosis, kidney degeneration, and cardiac arrhythmias, and possibly nasal lesions and immune-system depression. Exposures to lower concentrations, which do not cause liver or kidney pathology, can still cause cytotoxicity, as evidenced by increases in the labeling indices of these tissues. Long-term exposure to relatively high concentrations might lead to liver or kidney cancer.
Acute and Short-Term Exposures
Cardiac Effects
Chloroform anesthesia is associated with cardiac toxicity. In a 1965 epidemiological study by Whitaker and Jones (1965) of a cohort of 1502 patients (exposures at concentrations of 10,000 to 22,500 ppm), dose-related bradycardia developed in 8% of the cases, and cardiac arrhythmia developed in 1.3% of the cases. Hypotension was observed in 27% of the patients and was related to the duration of the anesthesia and to pretreatment with thiopentone. In 1973, Smith et al. reported that chloroform anesthesia (exposures at 8000 to 10,000 ppm) caused arrhythmia (nodal rhythm, first degree atrioventricular block, or complete heart block) in 50% of the cases from the cohort of 58 patients, and hypotension in 12% (Smith et al. 1973).
An EKG study of 66 patients anesthetized for at least 2 h at the State of Wisconsin General Hospital demonstrated that the effect of chloroform on the heart is not to induce ventricular fibrillation but rather depression of the myocardium to the point of asystole (Orth et al. 1951).
The EC50 (the concentration that induces a given affect in 50% of the exposed animals of a species in a given time) for sensitization to cardiac ar-
rhythmia in dogs exposed for 5 min to chloroform vapors was 16,000 ppm (Clark and Tinston 1982). This effect was rapidly reversed on cessation of exposure (Clark and Tinston 1982).
CNS
Evidence for chloroform's effects on the CNS come from occupational exposures and from the use in the past of chloroform as an inhalation anesthetic. The recommended method to induce anesthesia during surgery or childbirth involved increasing concentrations of chloroform gradually to 25,000 or 30,000 ppm during the first 2 or 3 min, with maintenance at much lower concentrations (ATSDR 1997). Concentrations < 1500 ppm are insufficient to induce anesthesia; 1500 to 2000 ppm causes light anesthesia (Goodman and Gilman 1980). Dizziness and vertigo occur after exposure to 920 ppm for 3 min; headache and slight intoxication occur at higher concentrations (Lehmann and Hasegawa 1910).
The EC50 for CNS depression (ataxia and loss of righting reflex) in rats exposed for 10 min to chloroform vapors was 16,000 ppm (Clark and Tinston 1982). That effect was rapidly reversed on cessation of exposure (Clark and Tinston 1982).
Hepatotoxicity
Several early studies reported acute hepatic necrosis in women exposed to chloroform via anesthesia (Royston 1924; Townsend 1939; Lunt 1953). The effects observed in women included jaundice, liver enlargement and tenderness, delirium, coma, and death. Centrilobular necrosis was found at autopsy in those who died. In 1973, Lieberman reported that there had not been any chloroform jaundice in 30,000 chloroform anesthesias at one hospital since 1942. Lieberman (1973) noted that other studies have documented hepatic necrosis due to all anesthetics, including cyclopropane, ether, ethylene, and nitrous oxide and reported a personal communication from a chief of pathology at a hospital that there had been no cases of chloroform hepatitis over a 15-y period from 1945 through 1960 during which 70,000 ''open-drop" chloroform anesthesias were administered for obstetrics. In the open-drop technique, chloroform was administered on a handkerchief. Until precision vaporizers became available in the late 1950s, anesthetists had no means to measure or control the concentration of chloroform in inspired air, and cases of hepatotoxicity were attributed
to overdosing by inexperienced anesthetists. Whitaker and Jones (1965) studied over 1500 patients receiving chloroform anesthesia administered by a precision vaporizer at nominal inspired concentrations of no greater than 22,500 ppm for durations of less than 30 min (1164 patients) to over 120 min (24 patients). The only case of hepatotoxicity found was transient jaundice in one patient 36 h after exposure to chloroform for 6 min, but the patient was believed to have been incubating infectious hepatitis before anesthesia.
Increased sulfobromophthalein retention was observed in some patients exposed to chloroform via anesthesia (exposures at 8000 to 10,000 ppm), indicating impaired liver function (Smith et al. 1973).
Brown et al. (1974) found that exposure of rats for 2 h to chloroform at 5000 or 10,000 ppm produced hepatic necrosis and destruction of microsomal enzymes. Treatment of rats with phenobarbital to induce microsomal enzyme activity before exposure to chloroform markedly increased the hepatotoxic response to anesthesia and produced a 70% to 80% decrease in hepatic glutathione concentrations. In rats in which microsomal enzyme activity was not induced with phenobarbital, chloroform exposure did not result in depletion of glutathione or in hepatic necrosis 24 h after exposure (Brown et al. 1974). Experimental depletion of hepatic glutathione by pretreatment with diethyl maleate also resulted in centrilobular necrosis after exposure to chloroform (Brown et al. 1974). These results suggest that much or all of the hepatotoxicity of chloroform is due to the production of reactive chloroform metabolites (e.g., phosgene).
Studies at the Chemical Industry Institute of Toxicology (CIIT) found large species-specific differences in the dose-related hepatotoxicity of chloroform inhaled 6 h/d for 7 d by B6C3F1 mice and Fischer 344 (F344) rats, with lowest-observed-adverse-effect levels (LOAELs) of 10 ppm and 300 ppm and no-observed-adverse-effect levels (NOAELs) of 3 ppm and 100 ppm, respectively (Larson et al. 1994b). They later tested for hepatotoxicity in male and female BDF1 mice exposed to chloroform for 6 h/d for 4 d at 0, 0.3, 5, 30, or 90 ppm or males exposed for 6 h/d, 5 d/w for 2 w at 0, 30, or 90 ppm (Templin et al. 1996a). Mild centrilobular hepatocyte vacuolization was seen in one of five male mice exposed at 30 ppm for 2 w, and centrilobular vacuolization and focal areas of hepatocyte necrosis were seen in three of four male mice and three of five female mice exposed at 90 ppm for 4 d. Significant increases in hepatocyte labeling index (LI) were seen in male mice exposed to chloroform at 30 and 90 ppm and in female mice exposed at 90 ppm (Templin et al. 1996a). Unpublished results from CIIT indicated that similar dose responses were obtained for increases in hepatocyte LIs in B6C3F1 mice exposed for periods of 7 d to 180 d (Butterworth 1997).
The observed species-specific differences in sensitivity to the hepatotoxic
effects of chloroform are not due to differences in the sensitivity of hepatocyte cells to the cytotoxic effects of chloroform or to differences in their ability to metabolize chloroform. When freshly isolated hepatocytes from B6C3F1 mice and F344 rats were exposed to solutions of chloroform for up to 3 h, concentration-dependent cytotoxicity (lactate dehydrogenase release) was seen in culture at concentrations higher than 1 mM (Ammann et al. 1998). Cotreatment with the cytochrome P-450 inhibitor 1-phenylimidazole prevented both cytolethality and glutathione depletion, indicating that metabolism is necessary for chloroform-induced cytotoxicity. These results correlate well with simulations of a physiologically based dosimetry model for chloroform. The simulations indicated that after hepatotoxic oral bolus doses of chloroform at 477 mg/kg of body weight, the livers of mice and rats were exposed to chloroform at concentrations up to 5 mM for 3 h (Ammann et al. 1998). Hepatocytes from the two species exhibited similar sensitivity toward chloroform toxicity, indicating that toxicity is not sufficient to explain different susceptibility to heptocarcinogenicity.
Lethality
Acute exposures to relatively high concentrations of chloroform can cause immediate death due to cardiovascular toxicity or delayed death (1 to 4 d after exposure) due to hepatotoxicity or nephrotoxicity.
In humans, obstetric use of chloroform anesthesia earlier in this century occasionally caused fatal toxicity (Royston 1924). Obstetric deaths occurred either during anesthesia, due to cardiac arrhythmias, or a few days after anesthesia, due to hepatotoxicity. A 1973 report by an anesthesiologist stated that there had been no deaths at one hospital in 30 y from over 30,000 chloroform anesthesias for obstetrics since 1942 (Lieberman 1973) and attributed previous reports of chloroform-related deaths to use by inexperienced anesthetists of the crude open-drop technique of administering chloroform on a handkerchief, which made it difficult to control exposure concentrations.
The LC50 (lethal concentration for 50% of the exposed animals) for rats exposed for 15 min to chloroform vapor was 76,000 ppm (Clark and Tinston 1982). For a 6-h exposure to chloroform vapors, the LC 50 was 1849 ppm in rats and 1260 ppm in mice (Bonnet et al. 1980).
Nausea
A frequent side effect of chloroform anesthesia (8000 to 22,500 ppm) was
nausea (Royston 1924; Townsend 1939; Whitaker and Jones 1965; Smith et al. 1973). Nausea was also reported by women employed in a lozenge factory while working in an atmosphere with chloroform concentrations ranging from 23 to 1163 ppm (average = 128 ppm) for a period of 3 to 10 y (Challen et al. 1958). Thirteen workers exposed to chloroform at >400 ppm for 1 to 5 mo and 18 workers exposed at 14.4 to 50.4 ppm for 1 to 4 mo developed nausea in association with toxic hepatitis (Phoon et al. 1983). The data available are insufficient to establish a NOAEL or LOAEL or a dose-response relationship for nausea.
Nephrotoxicity
Chloroform induces kidney toxicity, which, depending on the species and strain, can be more or less severe than the liver toxicity induced by the same dose. Reports of chloroform-induced kidney toxicity in humans are few and sketchy but always associated with severe liver toxicity. In case reports of women who died after exposure to chloroform anesthesia during childbirth, autopsy revealed fatty degeneration of the kidneys, indicating chloroform-induced damage (Royston 1924). Those deaths are most likely attributable to hepatotoxicity in fasted individuals rather than nephrotoxicity, because the same women were reported to have jaundice, liver enlargement and tenderness, and, at autopsy, centrilobular necrosis.
In laboratory animals, susceptibility to chloroform-induced nephrotoxicity varies greatly with species, strain, and sex. In BDF1 mice, Templin et al. (1996a) found degenerative lesions and an increase of 7- to 10-fold in the percentage of cells in the S phase in the kidneys of males, but not of females, inhaling chloroform at 30 or 90 ppm, 6 h/d, 5 d/w for 2 w. In males exposed for 2 w, 40% of the 30-ppm group and 80% of the 90-ppm group died with severe kidney damage, indicating that 30 and 90 ppm exceeded the maximum tolerated dose. The NOAEL for male BDF1 mice inhaling chloroform for 4 d, 6 h/d was 5 ppm. In contrast, B6C3F1 mice were more resistant to chloroform nephrotoxicity and had a NOAEL of 100 ppm for a 6-h/d, 7-d exposure (Larson et al. 1994b). Similarly exposed F344 rats had a LOAEL of 30 ppm and a NOAEL of 10 ppm for exposure-induced kidney-cell proliferation (Larson et al. 1994b).
In certain strains of mice, renal tubular necrosis was reported in 100% of males after exposure to chloroform at ≤ 240 ppm for 2 h (Derringer et al. 1953; Culliford and Hewitt 1957). Mice surviving the exposure were found to have tubular calcifications when examined 12 mo after exposure. The kidneys of female mice of the susceptible strains and of both male and female mice of other strains were completely unaffected. Females of the susceptible strains
became susceptible when treated with testosterone. Immature males and castrated males were resistant to chloroform nephrotoxicity (Culliford and Hewitt 1957). Although this phenomenon is scientifically interesting, it is not a good model for the susceptibility of humans to chloroform nephrotoxicity. Clinical experience with thousands of patients, both males and females, who have undergone chloroform anesthesia (exposure at 8000 to 22,000 ppm) suggests a low incidence of nephrotoxicity in humans (Whitaker and Jones 1965; Lieberman 1973; Smith et al. 1973).
Resistance to Respiratory Infection
Mice (five groups of approximately 30 mice per group per dose for each exposure duration) exposed to Threshold-Limit-Value (TLV) concentrations (10.6 ppm) of chloroform for 3 h/d for 5 d and simultaneously challenged with an aerosol of Streptococcus zooepidemicus had significantly increased mortality (43.7% compared with 30.4% for filtered-air Streptococcus controls) over a 14-d observation period (Aranyi et al. 1986). Alveolar macrophage function (percentage of bacteria killed in 3 h), however, was not depressed under those conditions. Mice similarly tested for only 1 d (3 h) had no significant increase in mortality (6.7% vs. 5.7% for controls) (Aranyi et al. 1986).
Subchronic and Chronic Exposures
Long-term inhalation of chloroform vapors has been shown to be toxic to the liver and kidneys of several species, including humans. Other reported adverse effects include species-specific nasal toxicity, CNS effects, and lethality due to pneumonia.
Carcinogenicity
There are no compelling reports of chloroform-induced cancers in humans, despite extensive human exposure due to its use in the past in industry, as an anesthetic, and as an ingredient in medicinals. Chloroform is the major byproduct of chlorination of drinking water, and several studies have implicated chlorination by-products in the etiology of specific cancers. A 1992 epidemiological report suggests that consumption of chlorination by-products in drinking water is associated with an increased risk of rectal and urinary bladder cancers (Morris et al. 1992). A 1997 epidemiological study found a clear dose-response
relationship between increasing chloroform concentrations in finished drinking water and an increased risk of colon cancer (Doyle et al. 1997). Increasing chloroform concentrations in treated water, however, are likely to be accompanied by parallel increases in the concentrations of other chlorination by-products. Those epidemiological studies cannot specify which of the chlorination by-products is responsible for the increased cancer incidence. Some of the other by-products of water chlorination (e.g., bromodichloromethane) are genotoxic, whereas chloroform is not.
In animal studies, high doses and dose rates of chloroform have been shown to induce cancer in the liver and kidneys of mice and rats. Most studies involved gavage dosing, although several recent studies have examined the effects of chloroform inhalation. The most commonly observed cancers involved the liver and kidney in mice and rats. Striking differences were observed in the organ specificity between species and between different strains of the same species.
Long-term inhalation of high doses of chloroform vapors has been shown to induce kidney cancer in male BDF1 mice (Yamamoto et al. 1994). In contrast, male and female F344 rats inhaling chloroform at 10, 30, or 90 ppm, 5 d/w for 2 y developed no tumors (Yamamoto et al. 1994). Studies at CIIT to elucidate the mechanisms for the lack of carcinogenicity in F344 rats found that under the conditions used in the Japanese bioassay, F344 rats showed only a marginal increase of cell proliferation in the kidneys of males and no exposure-induced histopathology or cell proliferation in the kidneys of females except at a highly toxic dose of 300 ppm, 7 d/w (Templin et al. 1996c). In BDF1 mice, however, cancer induction appeared to correlate with cytotoxicity. Chloroform was found to be cytotoxic to both the liver and the kidney of BDF1 and B6C3F1 mice, yet BDF1 mice develop kidney tumors but not liver tumors, and B6C3F1 mice develop liver tumors but not kidney tumors. From that, Templin et al. (1996a) concluded that induced toxicity and regenerative-cell proliferation are necessary but not sufficient to induce cancer in a given organ. In another study, Larson et al. (1996) found a NOAEL of 10 ppm for increases in the LI of liver cells in female and male B6C3F1 mice exposed to chloroform at 0, 0.3, 2, 10, 30, and 90 ppm, 6 h/d, 7d/w for up to 13 w and proposed that 10 ppm should also be a NOAEL for liver cancer in female B6C3F1 mice (Larson et al. 1996). In other words, chloroform carcinogenicity should have a threshold if tumorigenesis is dependent on regenerative-cell proliferation (Golden et al. 1997). That proposal was challenged by Melnick et al. (1998), who argued that tumors can be produced at low doses of other trihalomethanes that do not produce increases in LIs (i.e., tumorigenesis is not dependent on regenerative-cell proliferation at low doses), and they asserted that the same would be true of chloroform also (Melnick et al. 1998). The trihalomethanes that they used to support that
contention, however, are DNA reactive, whereas chloroform is not, thus weakening their argument.
Numerous animal studies have shown that chloroform can be carcinogenic when given orally. The ability to induce cancer varied with the species, strain, and sex of the exposed animals, with the rate (bolus vs. intermittent) at which the chloroform was delivered, and with the dosing vehicle.
Female Wistar rats exposed for a lifetime to chloroform in drinking water at an average concentration of 200 mg/kg/d had an increased incidence of hepatic neoplastic nodules, and lymphosarcoma was increased in males (Tumasonis et al. 1987). Male Osborne-Mendel rats exposed to chloroform in drinking water for a lifetime at 160 mg/kg/d had an increased incidence of kidney tubular-cell adenoma and carcinoma but those exposed at 81 mg/kg/d did not (Jorgenson et al. 1985). A time-weighted-average chloroform dose of 263 mg/kg/d in drinking water for 1-4 w did not increase the incidence of hepatocellular carcinomas and adenomas in female B6C3F1 mice (Jorgenson et al. 1985).
Male Osborne-Mendel rats exposed to chloroform at 90 mg/kg/d by gavage for 78 w developed kidney tubular-cell adenomas and carcinomas (NCI 1976). Sprague-Dawley rats, however, when exposed by gavage to chloroform in toothpaste at 60 and 165 mg/kg/d for 80 and 52 w, respectively, did not have an increased incidence of tumors (Palmer et al. 1979).
Dogs exposed to chloroform in toothpaste capsules at 30 mg/kg/d for 7.5 y had no increase in tumors (Heywood et al. 1979).
Mice exposed by gavage to chloroform in oil at 595 mg/kg/d for 30 d had an increased incidence of hepatomas, and those receiving 297 mg/kg/d did not (Eschenbrenner and Miller 1945). Female A/J mice exposed by gavage to chloroform in oil at 1800 mg/kg/d for 8 w had no increase in lung tumors (Stoner et al. 1986). Male B6C3F1 mice exposed to chloroform in drinking water at 257 mg/kg/d for 52 w had no increase in lung or liver tumors (Klaunig et al. 1986).
ICI mice chronically exposed to chloroform by gavage at 60 mg/kg/d had an increased incidence of kidney tumors, but those exposed at 17 mg/kg/d did not (Roe et al. 1979). The overall incidence of all tumors, however, was lower in mice receiving the highest dose of chloroform than in controls. No significant differences were seen in the incidence or severity of nephrotoxicity in mice with kidney tumors and those without tumors. Under the same conditions, C57B1, CBA, and CF/1 mice had no change in the frequency of tumors (Roe et al. 1979). Thus, the significance of the increased incidence of kidney tumors in ICI mice is questionable.
B6C3F1 mice exposed by gavage to chloroform in oil at ≥ 138 mg/kg/d for 78 w developed hepatocellular carcinomas (NCI 1976). B6C3F1 mice exposed to
chloroform in drinking water at 263 mg/kg/d for 2 y had no increase in tumor incidence (Jorgenson et al. 1985), nor did they have increased liver-cell proliferation after 4 d or 3 w of exposure at up to 1800 ppm (Larson et al. 1994a); those given chloroform in corn oil at 238 or 477 mg/kg had both centrilobular necrosis and markedly elevated regenerative-cell proliferation (Larson et al. 1994a). Those studies support the mechanistic-based idea that chloroform's carcinogenicity is dependent on its capacity to induce necrosis and regenerative-cell proliferation. For the liver, Larson et al. (1994a) proposed that "the most straightforward risk assessment for chloroform for this tissue would assign no increased cancer risk for dosing regimens that do not induce cytolethality and cell proliferation." The NOAEL for histopathological changes from exposure to chloroform given in corn oil was 10 mg/kg/d, and for induced cell proliferation, the NOAEL was 34 mg/kg/d (Larson et al. 1994a).
CNS Effects
Challen et al. (1958) reported on two groups of workers in a British lozenge factory occupationally exposed to chloroform vapors. One group of eight workers (four half-time and four full-time) was exposed for ~2 h/d for 3 to 10 y to chloroform vapors at 77 to 237 ppm and experienced depression, irritability, a feeling of being dazed, "slow witted," "slowness in grasping things," lack of concentration, lassitude at the end of the day, and a desire to sleep. Additional symptoms included flatulence, nausea, dry mouth, thirst, and frequent and scalding urination. All these symptoms could be present at work but were usually worse in the evening at home (all exposed employees stated that at times they were unable to concentrate on household duties), and symptoms often persisted to some extent during the weekend, suggesting that chloroform was gradually being released from accumulated stores in the body. The second group of nine workers was exposed for 2 h/d for 10-24 mo to chloroform vapors at 22 to 71 ppm and complained of lassitude, flatulence, and dryness of the mouth and throat. It was not stated how soon symptoms developed after a new employee began working in the chloroform-contaminated atmosphere. Unexposed control workers did not have any of these symptoms.
Li et al. (1993) reported a variety of neurobehavioral effects in workers occupationally exposed for 1 to 15 y to chloroform vapors. These effects included dizziness, fatigue, somnolence, insomnia, hypomnesia, anorexia, palpitation, increased scores for depression, anger, and fatigue, and adverse effects on neurobehavioral functions, including increased simple visual reaction time, digit-symbol substitution, digit span, Bentsen retention, and pursuit aiming. The concentration of chloroform in the breathing zones of the workers
ranged from 4.3 to 148 mg/m3 (0.87 to 30 ppm). The period of time over which the concentration measurements were taken was not stated, nor was any attempt made to temporally correlate effects in individuals with the exposure concentration at that time. The authors correlated the observed effects to the geometric means of the measured exposure concentrations (2.7 ppm and 5.9 ppm for two subgroups and 4.1 for the combined exposed groups). Because of the wide (>34-fold) variation in measured exposure concentrations, it is likely that the observed effects were seen only in individuals during exposure (for unknown durations) to concentrations at the high end of the range. Thus, it is inappropriate to ascribe the observed effects to the geometric mean of the measured concentrations.
Hepatotoxicity and Pneumonitis
There have been several reports of hepatotoxicity due to occupational exposures to chloroform vapors. A report of slight liver damage in factory workers exposed to chloroform at an average of 4.3 ppm for 1 to 15 y was based on a finding of increased serum concentrations of prealbumin and transferrin compared with 23 control workers (Li et al. 1993). Little weight is given to these results, however, because changes in serum concentrations of prealbumin and transferrin can be caused by many factors besides liver toxicity. In Singapore, 13 factory workers exposed to greater than chloroform at 400 ppm for 1 to 5 mo and 18 workers at another factory exposed at 14.4 to 50.4 ppm for 1 to 4 mo developed toxic hepatitis with symptoms, which included jaundice, nausea, and vomiting, without fever (Phoon et al. 1983). Those also tested negative for hepatitis B surface antigen. The total number of workers exposed to chloroform was not stated, but the department employing the 13 jaundiced workers had a total of 102 workers, and the factory employing the 18 jaundiced workers had a total of 360 employees in two departments, only one of which utilized chloroform. The measured exposure concentrations in these factories could therefore be considered LOAELs. In contrast, in a 1958 British study, relatively insensitive clinical tests for liver injury (thymol turbidity, serum bilirubin, and urine urobilinogen) gave negative or inconclusive results in two groups of workers at a lozenge factory exposed to chloroform at average concentrations of 71 to 237 ppm for 4 to 8 h/d for 3 to 10 y or 10 to 24 mo (Challen et al. 1958). Still another report of occupational chloroform-related hepatotoxicity was confounded by co-exposure to other solvents (Bomski et al. 1967).
Torkelson et al. (1976) exposed rats, guinea pigs, and rabbits to chloroform for 7 h/d, 5 d/w for 6 mo at 85, 50, or 25 ppm and dogs similarly at 25 ppm.
Additional groups of male rats were exposed for 4,2, or 1 h/d at 25 ppm. At 85 ppm, there were no adverse effects in male guinea pigs but marked microscopic pneumonitis in female guinea pigs. In rabbits exposed at 85 ppm, males had marked microscopic pneumonitis and foamy vacuolization and necrosis of the liver (Torkelson et al. 1976). Male rats exposed at 85 ppm had marked interstitial pneumonitis. Both male and female rats exhibited marked central lobular granular degeneration of the livers. At 50 ppm, no adverse effects were found in guinea pigs or rabbits; in rats, the effects were similar to but milder than those at 85 ppm. Female rats were affected less than males. At 25 ppm, male rats exposed for 7 h/d exhibited lobular granular degeneration with focal areas of necrosis throughout the liver. These effects were reversible within 6 W. No adverse effects were seen in male rats exposed to chloroform for 4, 2, or 1 h/d at 25 ppm. At 25 ppm, male guinea pigs showed microscopic granular degeneration and foamy vacuolization in the liver, and female guinea pigs showed foamy vacuolization centrally in the liver. Female rabbits showed slight microscopic changes in the lungs, liver, and kidneys.
In a recent study at CIIT, female B6C3F1 mice exposed to chloroform at 100 or 300 ppm, 6 h/d, for 7 d exhibited centrilobular hepatocyte necrosis and severe vacuolar degeneration of midzonal and periportal hepatocytes, and exposure to 10 or 30 ppm resulted in mild-to-moderate vacuolar changes in centrilobular hepatocytes (Larson et al. 1994b). Slight, dose-related increases in the hepatocyte nuclear labeling indices (LIs) were observed for exposure concentrations of 10 and 30 ppm, and the LIs were increased more than 30-fold in the 100and 300-ppm groups (Larson et al. 1994b). The CIIT study also reported that mild centrilobular degeneration was observed only in the livers of rats exposed at 300 ppm (Larson et al. 1994b).
Lethality
Torkelson et al. (1976) exposed rats, guinea pigs, and rabbits to chloroform at 85 or 50 ppm, 7 h/d, for 138 to 144 d or those species plus dogs at 25 ppm, 7, 4, 2, or 1 h/d for 126 to 132 exposures in 180 to 186 d. At 85 ppm, they found excess mortality attributed to pneumonia only in male rats. At 50 ppm, there was no excess mortality in any of the tested species.
Nasal Toxicity
No reports of nasal toxicity due to chloroform inhalation were found in the literature before 1994. A 1994 CIIT report stated that in the nasal passages of
male F344 rats, chloroform concentrations of 10 ppm and above (6 h/d, 7 d) induced histopathological changes that exhibited clear concentration-related severity (Larson et al. 1994b). These lesions consisted of respiratory epithelial goblet-cell hyperplasia and degeneration of Bowman's glands in olfactory mucosa, with an associated osseous hyperplasia of the endo-and ecto-turbinates in the periphery of the ethmoid region (Méry et al. 1994; Larson et al. 1994b). Similarly exposed female B6C3F1 mice did not exhibit such nasal lesions (Larson et al. 1994b). The only change noted in the nasal passages of exposed mice was increased cell proliferation without the osseous hyperplasia (Méry et al. 1994). In further studies at CIIT, edema and periosteal hypercellularity were observed in the nasal passages of Osborne-Mendel and F344 rats at single gavage doses of chloroform in corn oil at ≥ 90 mg/kg when necropsied 48 h after dosing (Templin et al. 1996b). No other studies were found that reported any nasal toxicity due to chloroform.
Nausea and Vomiting
Nausea and vomiting were reported in workers exposed to chloroform at 14 to 400 ppm for 1 to 6 mo (Phoon et al. 1983). Nausea as well as dry mouth and fullness of the stomach were reported by eight workers exposed to chloroform at 77 to 237 ppm for 3 to 10 y (Challen et al. 1958). Nausea and vomiting were commonly reported during or after chloroform anesthesia (Whitaker and Jones 1965).
Nephrotoxicity
The effects of chronic inhalation of chloroform vapors on the kidneys depends heavily on the sex, strain, and species being exposed as well as the exposure schedule. In a series of studies at CIIT, male F344 rats and male B6C3F1 mice exposed to chloroform vapors for 7 d/w for 13 w had LOAELs for kidney toxicity of 30 ppm, and female F344 rats and female B6C3F1 mice had NOAELs of 90 ppm (Larson et al. 1996; Templin et al. 1996c). When the exposure schedule was 5 d/w for 13 w, the LOAEL increased for male F344 rats from 30 to 90 ppm but decreased for male B6C3F1 mice from 30 to 10 ppm (Larson et al. 1996; Templin et al. 1996c). Male BDF1 mice exposed for 6 h/d for 4 d to chloroform at 0, 0.3, 5, 30, or 90 ppm had a LOAEL of 30 ppm and a NOAEL of 5 ppm for necrosis of the proximal convoluted tubules, tubule dilation, accumulation of hyaline casts, and focal mineralization of the kidneys (Templin et al. 1996a). Female BDF1 mice showed no kidney toxicity at any
tested dose up to 90 ppm. A NOAEL of 5 ppm for nephrotoxicity, cell proliferation, and cancer was demonstrated in BDF1 mice exposed for 2 y at concentrations of 5, 30, or 90 ppm for 6 h/d, 5 d/w (Templin et al. 1998).
Larson et al. (1994b) reported that about 25% to 50% of the proximal tubules were lined by regenerating epithelium in the kidneys of male F344 rats (females rats were not tested) and female B6C3F1 mice (male mice were not tested) inhaling chloroform at 300 ppm for 6 h/d for 7 d but not in those inhaling 100, 30. or 10 ppm (Larson et al. 1994b).
Torkelson et al. (1976) exposed rats, guinea pigs, and rabbits to chloroform for 7 h/d, 5 d/w for 6 mo at 85, 50, or 25 ppm and dogs similarly at 25 ppm. Additional groups of male rats were exposed for 4, 2, or 1 h/d at 25 ppm. In rabbits exposed at 85 ppm, females had cloudy swelling in the kidneys. Both male and female rats exhibited cloudy swelling of the kidneys at 85 ppm. At 50 ppm, no adverse effects were found in guinea pigs or rabbits; in rats, the effects were similar to but milder than those at 85 ppm. Female rats were affected less than males. At 25 ppm, male rats exposed for 7 h/d exhibited cloudy swelling of the renal tubular epithelium. Those effects were reversible within 6 w. No adverse effects were seen in male rats exposed for 4, 2, or 1 h/d. In female rats exposed to chloroform for 7 h/d at 25 ppm, the relative weights, but not the absolute weights, of kidney and spleen were significantly increased. All other measurements were normal. At 25 ppm, male guinea pigs showed interstitial and tubular nephritis in the kidneys, and female guinea pigs showed significantly higher absolute and relative kidney weights, contrary to what had been observed at higher concentrations. Rabbits showed only an increase of interstitial and tubular nephritis in males and slight microscopic changes in the lungs, liver, and kidneys in females. Male dogs exposed at 25 ppm showed no changes, but female dogs exhibited microscopic pathological changes in the kidneys.
Genotoxicity
No data were found on the genotoxicity in humans of inhaled chloroform vapor.
A number of laboratories have tested chloroform for mutagenicity in Salmonella and E. Coli using a wide range of concentrations with and without metabolic activation. Rosenthal (1987) critically reviewed these studies and, despite noting some deficiencies in experimental procedures, concluded that chloroform is not mutagenic in bacteria—a conclusion that the current literature still supports (Roldan-Arjona and Pueyo 1993; Pegram et al. 1997).
Tests of chloroform's mutagenicity in various eukaryotes have given mixed
results. Callen et al. (1980) obtained only marginal effects in yeast for mitotic gene conversion and crossing over, as well as gene reversion, at chloroform concentrations of 21, 41, and 54 mM for 1 h. Crebelli et al. (1988 1992) reported the induction of aneuploidy by a threshold concentration of 0.16% vol/vol in the fungus Aspergillus nidulans, but that result is questionable because aneuploidy was not found at 0.20%.
Sturrock (1977) found that chloroform did not cause mutations at the HGPRT locus in Chinese hamster lung fibroblasts exposed to a 1-2.5% solution for 24 h, but no metabolic activation was used. Both negative results (White et al. 1979; Kirkland et al. 1981) and positive results (Morimoto and Koizumi 1983) have been reported for induction of sister chromatid exchanges in human lymphocytes in vitro and mouse bone marrow in vivo, but some aspects of the procedures used preclude reaching definitive conclusions.
In a tightly controlled study of mutation at the thymidine kinase gene in the L5178Y TK+/- mouse lymphoma cell, Mitchell et al. (1988) reported mixed results in experiments with and without metabolic activation. The mutant colonies might have resulted from chromosome loss (aneuploidy).
No increase in lacI mutant frequency was seen in hepatocytes isolated from B6C3F1 lacI transgenic mice exposed by inhalation to chloroform at 0, 10, 30, or 90 ppm for 6 h/d, 7 d/w for up to 180 d (Butterworth et al. 1998).
No data have been reported that used tests recently designed for unequivocal detection of aneuploidy caused by chloroform, but, like other anesthetics, chloroform can disrupt the microtubules in the spindle of dividing cells. It is known that depolymerization of tubulin is involved in that action (see Liang et al. 1983), and low doses of depolymerizing agents can cause one or several chromosomes to come off the spindle, leading to aneuploid daughter cells. Chromosomes not attached to the spindle might form micronuclei, and that is probably the reason for the reports that chloroform causes small, statistically insignificant increases in micronuclei frequency, interpreted to be chromosomal aberrations (see Agustin and Lim-Sylianco 1978; Gocke et al. 1981). A 3.32-fold increase in the frequency of micronucleated kidney cells was reported by Robbiano et al. (1998) in male Sprague-Dawley rats given a single oral dose of 4 mmol/kg (476 mg/kg) of chloroform in corn oil.
Land et al. (1981) found statistically significant increases in the percentages of abnormal sperm heads in mice exposed to reagent grade chloroform for 4 h/d for 5 d at 400 or 800 ppm. That might be caused by one or more mutations, because abnormal sperm head shape in the mouse has been shown to be determined by genes. However, because cytoplasmic microtubules are still present in the early stages of sperm development (spermatids), abnormal sperm heads might alternatively be caused by depolymerized tubulin.
In summary, there are no convincing data that chloroform causes gene
mutation or chromosomal aberrations. It is more probable that it or its metabolic products act on proteins and not on DNA (Mersch-Sundermann et al. 1994) and are, therefore, likely to be aneugenic, but there are not yet definitive studies on that point. If it is an aneugen, it would have a concentration threshold for effect and would show a plateau at higher concentrations.
Reproductive Toxicity
No studies were found regarding reproductive effects in humans after inhalation exposure to chloroform vapors. An NTP study (Gulati et al. 1988) found that fertility was not affected in either of two generations of mice exposed by gavage to chloroform in corn oil at up to 41 mg/kg/d for 105 d, but at these doses, sperm morphology was not affected. In mice exposed by inhalation to chloroform at 400 or 800 ppm, 4 h/d for 5 d, Land et al. (1981) found statistically significant increases in the percentages of abnormal spermatozoa. In contrast, mice receiving five daily injections of chloroform intraperitoneally at 0.025, 0.05, 0.075, 0.1, and 0.25 mg/kg/d showed only nonreproducible, sporadic, small increases in abnormal sperm (Topham 1980).
Developmental and Fetal Toxicity
Embryotoxicity and fetotoxicity were found in pregnant Sprague-Dawley rats exposed to chloroform for 7 h/d at 100 or 300 ppm, but only minor embryo-and fetotoxicities were seen for exposure at 30 ppm on d 6 through 15 of gestation (Schwetz et al. 1974; Baeder and Hofmann 1988). A decreased ability to maintain pregnancy was observed in CF-1 mice exposed 7 h/d at 100 ppm on d 1 through 7 or 6 through 15, but no significant teratogenicity (Murray et al. 1979). When the exposure schedule was on d 8 through 15, however, no decrease was seen in the ability to maintain pregnancy, but a significant increase in the incidence of cleft palate was observed in the offspring.
No studies were found regarding developmental effects in humans after inhalation exposure to chloroform vapors. In rats exposed during gestation, chloroform-induced fetotoxicity and teratogenicity (decreased fetal crown-rump length and delayed ossification) were observed by Schwetz et al. (1974), but only at concentrations that produced maternal anorexia, with a LOAEL of 30 ppm. Murray et al. (1979) found increased incidences of cleft palate, decreased ossification, and decreased fetal crown-rump length in rats and an increased incidence of cleft palate in the offspring of mice exposed on d 8 through 15 of gestation to chloroform at 100 ppm.
Interaction with Other Chemicals
Chloroform-induced toxicity can be potentiated by several treatments. Some examples include ethanol, polybrominated biphenyls (PBBs), ketones, and steroids (EPA 1985). Chemicals, such as diethyl maleate, that deplete hepatic glutathione can greatly increase the hepatotoxicity of chloroform (Brown et al. 1974). Fasting, which also reduces glutathione, has a similar enhancing effect on chloroform hepatotoxicity (Brown et al. 1974).
Factors that appear to protect against toxicity include disulfiram and high carbohydrate diets (EPA 1985).
Table 13-1 presents a summary of the toxicity data on chloroform.
TABLE 13-1 Toxicity Summary
Concentration, ppm |
Exposure Duration |
Species |
Effects |
Reference |
Effects in Humans |
||||
2.7 |
1-15 y, occupational |
Human, n = 14 |
Dizziness, fatigue, somnolence, insomnia, hypomnesia, anorexia, palpitation, increased serum concentrations of transferrin, increased scores for depression, anger, and fatigue |
Li et al. 1993 |
5.9 |
1-15 y, occupational |
Human, n = 46 |
Dizziness, fatigue, somnolence, insomnia, hypomnesia, anorexia, palpitation; increased serum concentrations of prealbumin and transferrin; increased scores for depression, anger, and fatigue; adverse effects on neurobehavior (increased simple visual reaction time, digit-symbol substitution, digit span, Bentsen retention, and pursuit aiming) |
Li et al. 1993 |
10-200 |
1-4 y, occupational |
Human, n = 68 |
Hepatomegaly in 16 of 68 |
Bomski et al. 1967 |
14-400 |
1-6 mo, occupational |
Human, n = 31 |
Toxic hepatitis, jaundice, nausea, vomiting, no fever |
Phoon et al. 1983 |
22-71 |
10-24 mo, occupational |
Human, n = 10 |
Lassitude; no evidence of liver toxicity |
Challen et al. 1958 |
77-237 |
3-10 y, occupational |
Human, n = 10 |
No evidence of liver toxicity; lassitude, digestive disturbances, frequent burning urination, depression, lack of concentration, and irritability |
Challen et al. 1958 |
920 |
3 min |
Human |
Dizziness and vertigo |
Lehmann and Hasagawa 1910 |
1000 |
<1 h |
Human |
Dizziness, nausea, and after-effects of fatigue and headache |
Hathaway et al. 1991 |
<1500 |
NS |
Human |
NOEL for anesthesia |
Goodman and Gilman 1980 |
1500-2000 |
NS |
Human |
Light anesthesia |
Goodman and Gilman 1980 |
Concentration, ppm |
Exposure Duration |
Species |
Effects |
Reference |
Effects in Humans |
||||
4100 |
<1 h |
Human |
Serious disorientation |
Hathaway et al. 1991 |
8000-10,000 |
Anesthesia |
Human |
Increased sulfobromophthalein retention (impaired liver function); EC50 for anesthesia; cardiac arrhythmia in 50% of 58 patients |
Smith et al. 1973 |
10,000-22,500 |
Anesthesia |
Human |
Bradycardia (<60 beats/min) in 8% of 1502 patients; cardiac arrhythmia in 1.3% of 1,502 patients; hypotension in 27% of 1502 patients (related to pretreatment with thiopentone) |
Whitaker and Jones 1965 |
14,000-16,000 |
<1 h |
Human |
Rapid loss of consciousness |
Hathaway et al. 1991 |
20,000-40,000 |
<1 h |
Human |
Induction of anesthesia; continued exposure to 20,000 ppm results in respiratory failure, cardiac arrhythmia, and death |
Hathaway et al. 1991 |
Effects in Animals |
||||
2 |
6 h/d, 7 d/w, 13 w |
Rat, F-344 |
LOAEL for atrophy of nasal ethmoid turbinates |
Larson et al. 1996 |
3 |
6 h/d, 7 d |
Rat |
NOAEL for histopathological changes in nasal passages |
Larson et al. 1994b |
3 |
6 h/d, 7 d |
Mice, B6C3F1, female |
NOAEL for vacuolar changes in centrilobular hepatocytes, hepatocyte DNA synthesis/repair, nasal lesions |
Larson et al. 1994b |
5 |
6 h/d, 4 d |
Mice, BDF1, male and female |
NOAEL for hepatocyte vacuolization or necrosis |
Templin et al. 1996a |
5 |
6 h/d, 5 d + 4 d |
Mice, BDF1, male |
NOAEL for necrosis of the proximal convoluted tubules, tubule dilation, accumulation of hyaline casts, and focal mineralization of the kidneys |
Templin et al. 1996a |
10 |
6 h/d, 7 d |
Rat, F-344, male |
LOAEL for histopathological changes in nasal passages |
Méry et al. 1994 |
10 |
6 h/d, 7 d/w, 13 w |
Rat, F-344, male |
LOAEL for histopathological changes in nasal passages |
Larson et al. 1994b |
10 |
6 h/d, 7 d |
Rat, F-344, male |
NOAEL for increased LI in kidney |
Larson et al. 1994b |
Concentration, ppm |
Exposure Duration |
Species |
Effects |
Reference |
10 |
6 h/d, 7 d/w, 13 w |
Rat, F-344, male |
NOAEL for increased LI in kidney |
Larson et al. 1996 |
10.6 |
3 h |
Mice |
NOAEL for increased mortality with bacterial challenge |
Aranyi et al. 1986 |
10.6 |
3 h/d, 5 d |
Mice |
Increased mortality with bacterial challenge |
Aranyi et al. 1986 |
25 |
7 h/d, 5 d/w, 6 mo |
Rat, male |
Lobular granular degeneration with focal areas of necrosis of liver; cloudy swelling of renal tubular epithelium |
Torkelson et al. 1976 |
25 |
7 h/d, 5 d/w, 6 mo |
Rat, female |
Increased relative, but not absolute weights of kidney and spleen |
Torkelson et al. 1976 |
25 |
4 h/d, 5 d/w, 6 mo |
Rat, male |
NOAEL for liver, kidney, lung, organ weight, and blood effects |
Torkelson et al. 1976 |
25 |
7 h/d, 5 d/w, 6 mo |
Guinea pig, male |
Granular degeneration and vacuolization in the liver; interstitial and tubular nephritis |
Torkelson et al. 1976 |
25 |
7 h/d, 5 d/w, 6 mo |
Guinea pig, female |
Foamy vacuolization in the liver; increased absolute and relative kidney weights |
Torkelson et al. 1976 |
25 |
7 h/d, 5 d/w, 6 mo |
Rabbit, male |
Increased interstitial and tubular nephritis |
Torkelson et al. 1976 |
25 |
7 h/d, 5 d/w, 6 mo |
Rabbit, female |
Slight microscopic changes in the lungs, liver, and kidneys |
Torkelson et al. 1976 |
25 |
7 h/d, 5 d/w, 6 mo |
Dog, male |
NOAEL for liver, kidney, lung, organ weight, and blood effects |
Torkelson et al. 1976 |
25 |
7 h/d, 5 d/w, 6 mo. |
Dog, female |
Microscopic pathological changes in the kidneys |
Torkelson et al. 1976 |
Concentration, ppm |
Exposure Duration |
Species |
Effects |
Reference |
30 |
6 h/d, 2 w |
Mice, BDF1, male and female |
LOAEL for hepatocyte vacuolization |
Templin et al. 1996a |
30 |
6 h/d, 7 d/w, 90 d |
Rat, F-344 |
NOAEL for kidney cytotoxicity and regenerative-cell proliferation |
Templin et al. 1996c |
50 |
7 h/d, 5 d/w, 6 mo |
Guinea pig, rabbit |
NOAEL for liver, kidney, lung, organ weight, and blood effects |
Torkelson et al. 1976 |
50 |
7 h/d, 5 d/w, 6 mo |
Rat |
Cloudy swelling of kidney, granular degeneration of liver, LOAEL for weight loss |
Torkelson et al. 1976 |
75 |
4 h |
Rat, male |
NOAEL for increased serum concentrations of sorbitol dehydrogenase |
Lundberg et al. 1986 |
85 |
7 h/d, 5 d/w, 6 mo |
Rat, male |
Cloudy swelling of kidney, granular degeneration of liver, LOAEL for mortality due to interstitial pneumonitis |
Torkelson et al. 1976 |
85 |
7 h/d, 5 d/w, 6 mo |
Rat, female |
Cloudy swelling of kidney, granular degeneration of liver, NOAEL for interstitial pneumonitis |
Torkelson et al. 1976 |
85 |
4 h/d, 5 d/w, 6 mo |
Rat, male |
NOAEL for liver, kidney, lung, organ weight, blood effects |
Torkelson et al. 1976 |
90 |
6 h/d, 4 d |
Mice, BDF1, male and female |
LOAEL for hepatocyte vacuolization and necrosis and for increased hepatocyte LI in females |
Templin et al. 1996a |
90 |
6 h/d, 5 d/w, 2 y |
Rat, F344, male and female |
NOAEL for kidney cancer |
Yamamoto et al. 1994 |
90 |
6 h/d, 5 d/w, 90 d |
Rat, F-344, male and female |
LOAEL for kidney cytotoxicity and regenerative-cell proliferation in males |
Templin et al. 1996c |
Concentration, ppm |
Exposure Duration |
Species |
Effects |
Reference |
100 |
6 h/d, 7 d |
Mouse, B6C3F1, female |
NOAEL for kidney cytotoxicity |
Larson et al. 1994b |
100 |
6 h/d, 7 d |
Rat, F-344, male |
LOAEL for liver cytotoxicity |
Larson et al. 1994b |
100 |
6 h/d, 7 d/w, 13 w |
Rat, F-344, male |
NOAEL for hepatic lesions and increased LI |
Larson et al. 1996 |
100 |
7 h/d, 7-10 d |
Mouse |
Decreased ability to maintain pregnancy, increased incidence of cleft palate, decreased ossification, decreased incidence of resorptions, and reduced fetal body measurements |
Murray et al. 1979 |
100 |
7 h/d, 7-10 d |
Rat |
Retarded fetal development; low incidence of acaudate fetuses |
Murray et al. 1979 |
149 |
4 h |
Rat |
LOAEL for increased serum concentrations of sorbitol dehydrogenase |
Lundberg et al. 1986 |
300 |
6 h/d, 7 d |
Mouse, B6C3F1, female |
LOAEL for kidney cytotoxicity |
Larson et al. 1994b |
300 |
6 h/d, 7 d/w, 13 w |
Rat, F344 |
LOAEL for hepatic lesions and increased LI |
Larson et al. 1996 |
300 |
7 h/d, 10 d |
Rat |
Decreased incidence of pregnancy; increased incidence of resorptions |
Schwetz et al. 1974 |
400 |
4 h/d, 5 d |
Mouse |
Increased percentage of abnormal spermatozoa |
Land et al. 1981 |
1260 |
6 h |
Mouse |
LC50 |
Bonnet et al. 1980 |
1849 |
6 h |
Rat |
LC50 |
Bonnet et al. 1980 |
Concentration, ppm |
Exposure Duration |
Species |
Effects |
Reference |
1849 |
6 h |
Rat |
LC50 |
Bonnet et al. 1980 |
5000 |
2 h |
Rat |
Hepatic necrosis; destruction of microsomal enzymes |
Brown et al. 1974 |
9540 |
4 h |
Rat |
LC50 |
Lundberg et al. 1986 |
16,000 |
5 min |
Dog |
Sensitization to cardiac arrhythmia |
Clark and Tinston 1982 |
16,000 |
10 min |
Rat |
EC50 for ataxia and loss of righting reflex |
Clark and Tinston 1982 |
76,000 |
15 min |
Rat |
LC50 |
Clark and Tinston 1982 |
NS, not specified. |
RATIONALE FOR ACCEPTABLE CONCENTRATIONS
Table 13-2 presents exposure limits for chloroform set by other organization and Table 13-3 presents the SMACs established by NASA.
The SMAC values listed above were set based on the lowest acceptable
TABLE 13-2 Exposure Limits Set by Other Organizations
Organization |
Exposure Limit, ppm |
Reference |
ACGIH's TLV |
10 (TWA) |
ACGIH 1997 |
ACGIH's STEL |
Not set |
ACGIH 1997 |
OSHA's PEL |
2 (TWA) |
ACGIH 1991 |
NIOSH's REL |
2 (60-min ceiling) |
ACGIH 1991 |
NIOSH's IDLH |
1000 |
NIOSH 1990 |
NRC's 1-h EEGL |
100 |
NRC 1984 |
NRC's 24-h EEGL |
30 |
NRC 1984 |
NRC's 90-d CEGL |
1 |
NRC 1984 |
TLV, Theshold Limit Value; TWA, time-weighted average; STEL, short-term exposure limit; PEL, permissible exposure limit; REL, recommended exposure limit; IDLH, immediately dangerous to life and health; EEGL, emergency exposure guidance level; CEGL, continuous exposure guidance level. |
TABLE 13-3 Spacecraft Maximum Allowable Concentrations
Duration |
Concentration, ppm |
Concentration, mg/m3 |
Target Toxicity |
1 h |
2 |
10 |
CNS depression |
24 h |
2 |
10 |
CNS depression |
7 da |
2 |
10 |
CNS depression, hepatotoxicity, nephrotoxicity |
30 d |
1 |
5 |
CNS depression, hepatotoxicity |
180 d |
1 |
5 |
CNS depression, hepatotoxicity |
a Previous 7-d SMAC = 1 ppm. |
concentration (AC) among those calculated for the various adverse effects at each exposure duration, following the guidelines of the National Research Council (1992). The evidence and logic used to determine the ACs for each adverse effect and exposure duration are documented below. ACs were set for CNS effects, hepatotoxicity, nephrotoxicity, cardiac arrhythmia, and carcinogenicity (Table 13-4). No ACs were set for the following end points:
-
Nausea. Insufficient data are available to establish a dose-response relationship. In addition, it was seen in humans only in conjunction with CNS effects or hepatotoxicity.
-
Genotoxicity. There is no convincing evidence of chloroform's genotoxicity.
-
Resistance to respiratory infection. It is not clear to this author how to derive an AC for human exposures to chloroform under conditions of low level (ambient) bacterial challenge from the results reported by Aranyi et al. (1986). Attempts to use their data directly without adjustment for the massive bacterial challenge results in a 1-h AC of about 1 ppm (10.6 ppm NOAEL ÷ 10 (species)). Experience with human exposures to much higher concentrations for much longer exposures, including multi-year occupational exposures, has not produced any indication of increased susceptibility to respiratory infections, even when nausea and liver toxicity have been evident. No reports were found, however, that specifically looked for increases in respiratory infections.
-
Nasal lesions. Such lesions appear to be specific to rats and mice, and no nasal toxicity has ever been seen in humans or other animal species.
-
Developmental toxicity. Pregnant astronauts are not permitted to fly.
Carcinogenicity
The weight of evidence indicates that chloroform exposure results in tumors only under conditions resulting in exposure-induced cytotoxicity and cell regeneration (Golden et al. 1997), and even then, those effects appear to be necessary but not sufficient for tumorigenesis. The evidence from many studies supports the conclusion that chloroform exposures that do not produce cytotoxicity and cell regeneration will not result in tumorigenesis—a NOAEL for chloroform-induced cytotoxicity in a chloroform-sensitive species, strain, sex, and organ will be a NOAEL for carcinogenicity.
In humans, the organ most sensitive to chloroform toxicity appears to be the liver rather than the kidney. Despite numerous reports of chloroform-induced liver damage and even deaths in humans, there are no known reports of chloro-
form-induced liver cancer in humans, even those with substantial, multi-year occupational exposures. From that, one may surmise that it is likely that liver cytotoxicity and hepatocyte regeneration in humans is not sufficient to produce liver tumors. To err on the side of conservatism, however, the AC values will be derived assuming that humans might be sensitive to chloroform-induced hepatocarcinogenisis and basing them on NOAEL values for liver cytotoxicity in rodents inhaling chloroform vapors. A NOAEL for liver cancer can be deduced from the NOAEL of 10 ppm observed for liver cytotoxicity in female and male B6C3F1 mice exposed to chloroform at 0, 0.3, 2, 10, 30, and 90 ppm for 6 h/d, 7 d/w for up to 13 w (Larson et al. 1996). Because LI has not been found to increase with increasing exposure duration (Butterworth 1997), no adjustment is made for exposure duration. A species extrapolation factor of 1, rather than 10, is applied to the 10-ppm mouse NOAEL, because, at a given atmospheric concentration of chloroform vapor, the effective dose in the liver is lower in humans than in mice (Reitz et al. 1990) and because humans do not appear to be more sensitive than rodents to chloroform-induced carcinogenicity. No ACs are set for 1 h or 24 h exposures to carcinogens. Thus,
7-d, 30-d, and 180-d ACs = 10ppm ÷ 1 = 10ppm.
Cardiac Arrhythmia
An AC can be set based on the EC50 of 8000 ppm reported by Smith et al. (1973) for arrhythmia in patients receiving chloroform anesthesia. The EC50 is divided by 10 to estimate a NOAEL and divided again by an additional factor of 5 for possible spaceflight-enhanced sensitivity to noncritical arrhythmias. Thus,
AC = 8000 ppm (EC50) ÷ 10 (to NOAEL) ÷ 5 (spaceflight) = 160 ppm.
Because this effect is dependent only on the concentration of chloroform, is inducible within the first few minutes of exposure and is rapidly reversible on cessation of exposure, a concentration that would not induce arrhythmia for a 1-h exposure would also not induce arrhythmia for exposures of longer duration. Thus, the AC would apply for all exposure durations.
CNS Depression
ACs for CNS depression can be based on the estimated LOAEL of 22 ppm in workers reported by Challen et al. (1958). A 10-fold factor is applied to
estimate a NOAEL. Because CNS effects of solvents are generally dependent on blood concentration and independent of exposure duration, the calculated AC is applicable for all exposure durations ≤ 1 h. Although mild effects, such as irritation, which would not compromise a crew member's ability to safely perform his duties, might be tolerable for exposures of 24 h or less, mild CNS depression could affect a crew member's judgment and reaction time and thus would compromise safety and would be unacceptable, even for short exposures. Thus, for all exposure durations,
AC = 22 ppm ÷ 10 = 2 ppm.
Hepatotoxicity
No data are available to calculate an AC for 1 h or 24 h.
ACs for 7 d and 30 d can be calculated from the data of Phoon et al. (1983) for jaundice in 18 workers exposed to chloroform at an average of 32 ppm for 1 to 4 mo for an unstated number of hours per week; 8 of the cases occurred within the first month of exposure. Typical work schedules for such industries in Singapore during that time involved at least 8 h/d for 6 d/w. Using 32 ppm as a LOAEL for exposures of 206 h/mo (8 h/d × 6 d/w × 4.3 w/mo), a 7-d NOAEL is estimated by applying a safety factor of 10 to the 1-mo LOAEL. Thus,
7-d AC = 32 ppm ÷ 10 (to NOAEL) = 3 ppm.
A 30-d AC is calculated similarly, adjusting for the duration of exposure.

In a 1958 study, 17 workers in a lozenge factory exposed occupationally to chloroform at 23 to 71 ppm for 10 to 24 mo showed minimal evidence of liver toxicity. The tests used, however, were rather insensitive, so minor liver damage cannot be excluded. Because the exposures produced CNS and gastrointestinal-tract symptoms, which often precede symptoms of hepatotoxicity, 22 ppm will be treated as a LOAEL for subclinical hepatotoxicity for 4-h/d exposures up to 24 mo. The 180-d AC is set at the human LOAEL of 22 ppm, divided by 10 to estimate a NOAEL, and adjusted for exposure duration.
Thus,

Nephrotoxicity
ACs for nephrotoxicity are set based on data from animal experiments because few or no data exist on the nephrotoxic effects of inhalation exposures to chloroform in humans other than autopsy reports on women who died as a result of chloroform anesthesia during childbirth. Those reports found fatty degeneration of kidneys believed to be due to chloroform exposure in some of the patients (Royston 1924), but the exposure concentrations that produced such toxicity were not reported.
In males of the most sensitive species of mice, Templin et al. (1996a) found an experimental NOAEL of 5 ppm for kidney necrosis after exposures of 6-h/d for 4 d or for 2 w (5 d + 4 d). Their NOAEL is used to derive an AC for nephrotoxicity. A species extrapolation factor of 1 is used because PBPK modeling of the rate at which inhaled chloroform reaches the liver shows that, at any given atmospheric concentration, humans will achieve lower hepatic (and, by extension, kidney) chloroform concentrations than will mice. In addition, the data available suggest that the susceptibility of human tissues to injury by the active metabolites of chloroform does not exceed that of mouse tissues (Reitz et al. 1990). The total exposure time in the Templin et al. (1996a) experiment was 54 h, (6 h/d × 9 d). Thus, for exposure durations shorter than 54 h, (i.e., 1 h and 24 h), the ACs are set equal to the 5-ppm NOAEL, and for the 7-d AC, the NOAEL is adjusted for exposure duration using Haber's rule. Thus,
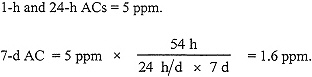
No 30-d or 180-d AC was set using these data because they would require a time extrapolation of greater than 10-fold.
Reproductive and Developmental Toxicity
ACs are not calculated for chloroform's ability to cause changes in sperm morphology, because Land et al. (1981) stated that the clinical significance of their findings (up to 3.5% abnormal sperm in mice exposed 4 h/d for 5 d at 800 ppm) cannot be evaluated because they did not study mating outcomes. ACs are also not calculated for decreased ability to maintain pregnancy, decreased conception rates in females, or teratogenic effects because NASA policy does not permit pregnant astronauts to fly.
Spaceflight Effects
Spaceflight is believed to increase the susceptibility of crew members to noncritical cardiac arrhythmias and could amplify the arrhythmogenic effects of chloroform.
TABLE 13-4 Acceptable Concentrations
End Point, Exposure Data, Reference |
|
Uncertainty Factors |
Acceptable Concentrations, ppm |
|||||||
Species |
NOAEL |
Species |
Time |
Spaceflight |
1 h |
24 h |
7 d |
30 d |
180 d |
|
Carcinogenicity (liver cytotoxicity) |
Mouse |
1 |
1 |
1 |
1 |
NS |
NS |
10 |
10 |
10 |
NOAEL, 10 ppm (Larson et al. 1996) |
|
|
|
|
|
|
|
|
|
|
Cardiac arrhythmia |
Human |
10 |
1 |
1 |
5 |
160 |
160 |
160 |
160 |
160 |
8000 ppm = EC50 (Smith et al. 1973) |
|
|
|
|
|
|
|
|
|
|
CNS depression |
Human |
10 |
1 |
1 |
1 |
2 |
2 |
2 |
2 |
2 |
LOAEL, 22 ppm (Challen et al. 1958) |
|
|
|
|
|
|
|
|
|
|
Hepatotoxicity |
Human |
10 |
1 |
HR |
1 |
NS |
NS |
3 |
1 |
NS |
LOAEL 32 ppm, 8 h/d, 6 d/w, 1 mo (Phoon et al. 1983) |
|
|
|
|
|
|
|
|
|
|
LOAEL, 22 ppm, 4 h/d, 5 d/w for 24 mo (Challen et al. 1958) |
Human |
10 |
1 |
HR |
1 |
NS |
NS |
NS |
NS |
1 |
Nephrotoxicity |
Mouse, BDF1, male |
1 |
1 |
1 |
1 |
5 |
5 |
1.6 |
NS |
NS |
NOAEL increases in LI, 5 ppm, 6 h/d, 4 d (Templin et al. 1996a) |
|
|
|
|
|
|
|
|
|
|
SMACs |
|
|
|
|
|
2 |
2 |
2 |
2 |
1 |
—, not applicable; NS, not set; HR, Haber's rule. |
RECOMMENDATIONS
Because chloroform's toxicity to the liver, kidney, and possibly the respiratory tract is due to its metabolites produced by cytochrome P-450, research is needed to quantitate the organ-specific levels of metabolism in humans and to elucidate factors, such as glutathione concentrations, which could modulate the threshold concentration of chloroform required for toxicity. Once those are determined, a PBPK model incorporating these values would be useful.
REFERENCES
ACGIH. 1991. Chloroform. Pp. 289-291 in Documentation of the Threshold Limit Values and Biological Exposure Indices, Vol 1, 6th Ed. American Conference of Governmental Industrial Hygienists, Cincinnati, OH.
ACGIH. 1991. Guide to Occupational Exposure Values—1991. American Conference of Governmental Industrial Hygienists, Cincinnati, OH.
ACGIH. 1997. P. 19 in 1997 TLVs and BEIs. American Conference of Governmental Industrial Hygienists, Cincinnati, OH.
Agustin, J.S., and C.Y. Lim-Sylianco. 1978. Mutagenic and clastogenic effects of chloroform. Bull. Phil. Biochem. Soc. 1:17-23.
Ahmed, A.E., V.L. Kubic and M.W. Anders. 1977. Metabolism of haloforms to carbon monoxide. I. In vitro studies . Drug Metab. Dispos. 5:198-204.
Ammann, P., C.L. Laethem, and G.L. Kedderis. 1998. Chloroform-induced cytolethality in freshly isolated male B6C3F1 mouse and F-344 rat hepatocytes. Toxicol. Appl. Pharmacol. 149:217-225.
Anders, M.W., J.L. Stevens, R.W. Sprague, Z. Shaath, and A.E. Ahmed. 1978. Metabolism of haloforms to carbon monoxide. II. In vivo studies. Drug Metabol. Dispos. 6:556-560.
Aranyi, C., W.J. O'Shea, J.A. Graham, and F.J. Miller. 1986. The effects of inhalation of organic chemical air contaminants on murine lung host defenses. Fundam. Appl. Toxicol. 6:713-720.
ATSDR. 1997. Toxicological Profile for Chloroform. TP-92-07. U.S. Department of Health and Human Services, Agency for Toxic Substances and Disease Registry, Atlanta, GA.
Baeder, C., and T. Hofmann. 1988. Inhalation Embryotoxicity Study of Chloroform in Wistar Rats. Frankfurt: Pharma Research Toxicology and Pathology, Hoechst Aktiengesellschaft.
Bomski, H., A. Sobolewska, and A. Strakowski. 1967. Toxic damage of the liver by chloroform in chemical industry workers. Int. Arch. F. Gewerbepathol. Gewerbehyg. 24:127-134.
Bonnet, P., J.M. Francin, D. Gradiski, G. Raoult, and D. Zissu. 1980. Determination
of the median lethal concentration of principal chlorinated aliphatic hydrocarbons in the rat. Arch. Mal. Prof. Med. Trav. Secur. Soc. 41:317-321.
Branchflower, R.V., D.S. Nunn, R.J. Highest, J.H. Smith, J.B. Hook, and L.R. Pohl. 1984. Nephrotoxicity of chloroform: Metabolism to phosgene by the mouse kidney. Toxicol. Appl. Pharmacol. 72:159-168.
Brown, B.R., Jr., I.G. Sipes, and A.M. Sagalyn. 1974. Mechanisms of acute hepatic toxicity: Chloroform, halothane, and glutathione. Anesthesiology 41:554-561.
Brown, D.M., P.F. Langley, D. Smith, and D.C. Taylor. 1974. Metabolism of chloroform—I. The metabolism of [14C]-chloroform by different species. Xenobiotica 4:151-163.
Butterworth, B.E., M.V. Templin, A.A. Constan, C.S. Sprankle, B.A. Wong, L.J. Pluta, J.I. Everitt, and L. Recio. 1998. Long-term mutagenicity studies with chloroform and dimethylnitrosamine in female lacI transgenic B6C3F1 mice. Environ. Mol. Mutagen. 31:248-256.
Callen, D.F., C.R. Wolf, and R.M. Philpot. 1980. Cytochrome P-450 mediated genetic activity and cytotoxicity of seven halogenated aliphatic hydrocarbons in Saccharomyces cerevisiae. Mutat. Res. 77:55-63.
Challen, P.J.R., D.E. Hickish, and J. Bedford. 1958. Chronic chloroform intoxication. Br. J. Ind. Med. 15:243-249.
Chiou, W.L. 1975. Quantitation of hepatic and pulmonary first-pass effect and its implications in pharmacokinetic study. I. Pharmacokinetics of chloroform in man. J. Pharmacokinet. Biopharm. 3:193-201.
Clark, D.G., and D.J. Tinston. 1982. Acute inhalation toxicity of some halogenated and nonhalogenated hydrocarbons. Hum. Toxicol. 1:239-247.
Cohen, E.N. 1971. Metabolism of the volatile anesthetics. Anesthesiology 35:193-202.
Corley, R.A., A.L. Mendrala, F.A. Smith, D.A. Staats, M.L. Gargas, R.B. Conolly, M.E. Andersen, and R.H. Reitz. 1990. Development of a physiologically based pharmacokinetic model for chloroform. Toxicol. Appl. Pharmacol. 103:512-527.
Crebelli, R., C. Andreoli, A. Carere, G. Conti, M. Cotta-Ramusino, and R. Benigni. 1992. Induction of chromosome malsegregation by halogenated organic solvents in Aspergillus nidulans: Quantitative structure activity relationship (QSAR) analysis with chlorinated aliphatic hydrocarbons. Mutat. Res. 266:117-134.
Crebelli, R., R. Benigni, J. Franekic, G. Conti, L. Conti, and A. Carere. 1988. Induction of chromosome malsegregation by halogenated organic solvents in Aspergillus nidulans: Unspecified or specified mechanism? Mutat. Res. 201:401-411.
Culliford, D., and H.B. Hewitt. 1957. The influence of sex hormone status on the susceptibility of mice to chloroform-induced necrosis of the renal tubules. J. Endocrinol. 14:381-393.
DeGroot, H., and T. Noll. 1989. Halomethane hepatotoxicity: Induction of lipid peroxidation and inactivation of cytochrome P-450 in rat liver microsomes under low oxygen partial pressures. Toxicol. Appl. Pharmacol. 97:530-537.
Derringer, M.K., T.B. Dunn and W.E. Heston. 1953. Results of exposure of strain C3H mice to chloroform. Proc. Soc. Exp. Biol. Med. 83:474-479.
Dix, K.J., G.L. Kedderis, and S.J. Borghoff. 1997. Vehicle-dependent oral absorption
and target tissue dosimetry of chloroform in male rats and female mice. Toxicol. Lett. 91:197-209.
Docks, E.L., and G. Krishna. 1976. The role of glutathione in chloroform-induced hepatotoxicity. Exp. Mol. Pathol. 24:13-22.
Doyle, T.J., W. Zheng, J. R. Cerhan, C.-P. Hong, T.A. Sellers, L.H. Kushi, and A.R. Folsom. 1997. The association of drinking water source and chlorination by-products with cancer incidence among postmenopausal women in Iowa: A prospective cohort study. Am. J. Public Health 87:1168-1176.
EPA. 1985. Health Assessment Document for Chloroform. PB86-105004. U.S. Environmental Protection Agency, Washington, DC. NTIS Publ. Doc. PB86-105004.
Eschenbrenner, A.B., and E. Miller. 1945. Induction of hepatomas in mice by repeated oral administration of chloroform, with observations on sex differences. J. Natl. Cancer Inst. 5:251-255.
Fry, B.J., R. Taylor, and D.E. Hathaway. 1972. Pulmonary elimination of chloroform and its metabolite in man. Arch. Int. Pharmacodyn.Ther. 196:98-111.
Gocke, E., M.T. King, K. Eckhardt, and D. Wild. 1981. Mutagenicity of cosmetics ingredients licensed by the European Communities. Mutat. Res. 90:91-109.
Golden, R.J., S.E. Holm, D.E. Robinson, P.H. Julkunen, and E.A. Reese. 1997. Chloroform mode of action: Implications for cancer risk assessment. Regul. Toxicol. Pharmacol. 26:142-155.
Gomez, M.I.D., and J.A. Castro. 1980. Nuclear activation of carbon tetrachloride and chloroform. Res. Commun. Chem. Pathol. Pharmacol. 27:191-194.
Goodman, L.S., and A. Gilman, eds. 1980. The Pharmacological Basis of Therapeutics. New York: Macmillan.
Gordon, S.M., L.A. Wallace, E.D. Pellizzari, and H.J. O'Neill. 1988. Human breath measurements in a clean-air chamber to determine half-lives for volatile organic compounds. Atmos. Environ. 22:2165-2170.
Guastedisegni, C., L. Guidoni, M. Balduzzi, V. Viti, E. DiConsiglio, and L. Vittozi. 1998. Characterization of a phospholipid adduct formed in Sprague Dawley rats by chloroform metabolism: NMR studies. J. Biochem. Mol. Toxicol. 12:93-102.
Gulati, D.K., E. Hope, R.C. Mounce, S. Russell, and K.B. Poonacha. 1988. Chloroform: Reproduction and fertility assessment in CD-1 mice when administered by gavage. NTP 89-018; PB89-148639. Prepared by Environmental Health Research and Testing, Inc., Lexington, KY, for National Toxicology Program, Research Triangle Park, NC
Hathaway, G.J., N.H. Proctor, J.P. Hughes, and M.L. Fishman, eds. 1991. Chloroform Pp. 168-169 in Proctor and Hughes' Chemical Hazards of the Workplace, 3rd Ed. New York: Van Nostrand Reinhold.
Heywood, R., R.J. Sortwell, P.R.B. Noel, A.E. Street, D.E. Prentice, F.J.C. Roe, P.F. Wadsworth, A.N. Worden, and N.J. Van Abbe. 1979. Safety evaluation of toothpaste containing chloroform. III. Long-term study in beagle dogs. J. Environ. Pathol. Toxicol. 2:835-851.
Huntoon, C.L. 1987. Introduction Summary Report of Postflight Atmospheric Analysis
for STS-41-D to 61-C. SD4/87-253. National Aeronautics and Space Administration, Lyndon B. Johnson Space Center, Houston, TX.
Huntoon, C.L. 1993. Summary Report of Preflight and Postflight Atmospheric Analyses for STS-26 through STS-41. SD4-93-021. National Aeronautics and Space Administration, Lyndon B. Johnson Space Center, Houston, TX.
James, J.T., T.F. Limero, H J. Leaño, J.F. Boyd, and P.A. Covington. 1994. Volatile organic contaminants found in the habitable environment of the Space Shuttle: STS-26 to STS-55. Aviat. Space Environ. Med 65:851-857.
Jorgenson, T.A., E.F. Meierhenry, C.J. Rushbrook, R.J. Bull, and M. Robinson. 1985. Carcinogenicity of chloroform in drinking water to male Osborne-Mendel rats and female B6C3F1 mice. Fundam. Appl. Toxicol. 5:760-769.
Kirkland, D.J., K.L. Smith, and N.J. Van Abbe. 1981. Failure of chloroform to induce chromosome damage or sister chromosome exchanges in cultured human lymphocytes and failure to induce reversion in Escherichia coli. Food Cosmet. Toxicol. 19:651-656.
Klaunig, J.E., R.J. Ruch, and M.A. Periera. 1986. Carcinogenicity of chlorinated methane and ethane compounds administered in drinking water to mice. Environ. Health Perspect. 69:89-95.
Land, P.C., E.L. Owen, and H.W. Linde. 1981. Morphological changes in mouse spermatozoa after exposure to inhalational anesthetics during early spermatogenesis. Anesthesiology 54:53-56.
Larson, J.L., M.V. Templin, D.C. Wolf, K.C. Jamison, J.R. Leininger, S. Méry, K.T. Morgan, B.A. Wong, R.B. Conolly, and B.E. Butterworth. 1996. A 90-day chloroform inhalation study in female and male B6C3F 1 mice: Implications for cancer risk assessment. Fundam. Appl. Toxicol. 30:118-137.
Larson, J.L., D.C. Wolf, and B.E. Butterworth. 1994a. Induced cytolethality and regenerative cell proliferation in the livers and kidneys of male B6C3F1 mice given chloroform by gavage. Fundam. Appl. Toxicol. 23:537-543.
Larson, J.L., D.C. Wolf, K.T. Morgan, S. Méry, and B.E. Butterworth. 1994b. The toxicity of 1-week exposures to inhaled chloroform in female B6C3F1 mice and male F-344 rats. Fundam. Appl. Toxicol. 22:431-446.
Lehmann, K.B., and D. Hasegawa. 1910. Absorption of chlorinated hydrocarbon compounds from the air in animals and man. Arch. Hyg. 72:327-342.
Li, L.H., X.Z. Jiang, Y.X. Liang, .Z.Q. Chen, Y.F. Zhou, and Y.L. Wang. 1993. Studies on the toxicity and maximum allowable concentration of chloroform. Biomed. Environ. Sci. 6:179-186.
Liang, J.C., T.C. Hsu, and J.E. Henry. 1983. Cytogenetic assays for mitotic poisons: The grasshopper embryo system for volatile liquids. Mutat. Res. 113:467-479.
Lieberman, S.L. 1973. Chloroform anesthesia. Anesth. Analg. 52:673-675.
Lundberg, I., M. Ekdahl, T. Kronevi, V. Lidums, and S. Lundberg. 1986. Relative hepatotoxicity of some industrial solvents after intraperitoneal injection or inhalation exposure in rats. Environ. Res. 40(2):411-420.
Lunt, R.L. 1953. Delayed chloroform poisoning in obstetric practice. Br. Med. J. 1:489-490.
McCarty, L.P., R.S. Malek, and E.R. Larsen. 1979. The effects of deuteration on the metabolism of halogenated anesthetics in the rat. Anesthesiology 51:106-110.
Melnick, R.L., M.C. Kohn, J.K. Dunnick, and J.R. Leininger. 1998. Regenerative hyperplasia is not required for liver tumor induction in female B6C3F1 mice exposed to trihalomethanes. Toxicol. Appl. Pharmacol. 148:137-147.
Mersch-Sundermann, V., U. Schneider, G. Klopman, and H.S. Rosenkranz. 1994. SOS induction in Eschericia coli and Salmonella mutagenicity: A comparison using 330 compounds. Mutagenesis 9:205-224.
Mery, S., J.L. Larson, B. E. Butterworth, D. C. Wolf, R. Harden, and K. T. Morgan. 1994. Nasal toxicity of chloroform in male F-344 rats and female B6C3F1 mice following a 1-week inhalation exposure. Toxicol. Appl. Pharmacol. 125:214-227.
Mitchell, A.D., B.C. Myhr, C.J. Rudd, W.J. Caspary, and V.C. Dunkel. 1988. Evaluation of the L5178Y mouse lymphoma cell system: Methods used and chemicals evaluated. Environ. Mol. Mutagen. 12 (Suppl 13):1-18.
Morimoto, K., and A. Koizumi. 1983. Trihalomethanes induce sister chromatid exchanges in human lymphocytes in vitro and mouse bone marrow cells in vivo. Environ. Res. 32:72-79.
Morris, R.D., A.M. Audet, I.F. Angelillo, T.C. Chalmers, and F. Mosteller. 1992. Chlorination, chlorination by-products, and cancer: A meta-analysis. Am. J. Public Health 82:955-963.
Murray, F.J., B.A. Schwetz, J.G. McBride, and R.E. Staples. 1979. Toxicity of inhaled chloroform in pregnant mice and their offspring. Toxicol. Appl. Pharmacol. 50:515-522.
NCI. 1976. Report on Carcinogenesis Bioassay of Chloroform. Carcinogenesis Program, Division of Cancer Cause and Prevention, National Cancer Institute, Bethesda, MD.
NIOSH. 1990. NIOSH Pocket Guide to Chemical Hazards. Publication No. 90-117 . National Institute for Occupational Safety and Health, Cincinnati, OH.
NRC. 1984. Chloroform. Pp. 57-76 in Emergency and Continuous Exposure Limits for Selected Airborne Contaminants, Vol. 1. Washington, DC: National Academy Press.
NRC. 1992. Guidelines for Developing Spacecraft Maximum Allowable Concentrations for Space Station Contaminants. Washington, DC: National Academy Press.
Orth, O.S., R.R. Liebenow, and R.T. Capps. 1951. The Effect of Chloroform on the Cardiovascular System. Pp. 39-75 in Chloroform. A Study after 100 Years, R.M. Waters, ed. Madison: University of Wisconsin Press.
Palmer, A.K., A.E. Street, J.C. Roe, A.N. Worden, and N.J.V. Abbé. 1979. Safety evaluation of toothpaste containing chloroform. II. Long term studies in rats. J. Environ. Pathol. Toxicol. 2:821-833.
Pegram, R.A., M.E. Anderson, S.H. Warren, T.M. Ross, and L.D. Claxton. 1997. Glutathione S-transferase-mediated mutagenicity of trihalomethanes in Salmonella typhimurium: Contrasting results with bromodichloromethane and chloroform. Toxicol. Appl. Phamacol. 144:183-188.
Phoon, W.H., K.T. Goh, and L.T. Lee. 1983. Toxic jaundice from occupational
exposure to chloroform
. Med. J. Malaysia 38:31-34.Pohl, L.R., B. Bhooshan, N.F. Whittaker, and G. Krishna. 1977. Phosgene: A metabolite of chloroform. Biochem. Biophys. Res. Commun. 79:684-691.
Pohl, L. R., ed. 1979. Biochemical toxicology of chloroform. Rev. Biochem. Toxicol. 1:79-107.
Pohl, L. R., J.L. Martin, A.M. Taburet, and J.W. George. 1980. Oxidative bioactivation of haloforms into hepatotoxins. Microsomes Drug Oxid. Chem. Carcinog. 2:881-884.
Raabe, O.G. 1988. Inhalation Uptake of Xenobiotic Vapors by People. ARB-R-88/338. 94 pp. Gov. Rep. Announce. Index (U.S.) 1988, 88(16), Abstr. No. 842, 087. University of California at Davis. NTIS Publ. Doc. PB88-202726.
Reitz, R.H., A.L. Mendrala, R.A. Corley, J.F. Quast, M.L. Gargas, M.E. Andersen, D.A. Staats, and R.B. Conolly. 1990. Estimating the risk of liver cancer associated with human exposures to chloroform using physiologically based pharmacokinetic modeling. Toxicol. Appl. Pharmacol. 105:443-459.
Robbiano, L., E. Mereto, A. Migliazzi Morando, P. Pastore, and G. Brambilla. 1998. Increased frequency of micronucleated kidney cells in rats exposed to halogenated anesthetics. Mutat. Res. 413:1-6.
Roe, F.J.C., A.K. Palmer, and A.N. Worden. 1979. Safety evaluation of toothpaste containing chloroform. I. Long-term studies in mice. J. Environ. Pathol. Toxicol. 2:799-819.
Roldan-Arjona, T., and C. Pueyo. 1993. Mutagenic and lethal effects of halogenated methanes in the Ara test of Salmonella typhimurium: Quantitative relationship with chemical reactivity. Mutagenesis 8:127-131.
Rosenthal, S.L. 1987. A review of the mutagenicity of chloroform. Environ. Mol. Mutagen. 10:211-226.
Royston, G.D. 1924. Delayed chloroform poisoning following delivery. Am. J. Obstet. Gynecol. 10:808-814.
Schwetz, B.A., B.K.J. Leong, and P.J. Gehring. 1974. Embryo-and fetotoxicity of inhaled chloroform in rats. Toxicol. Appl. Pharmacol. 28:442-451.
Sipes, I.G., G. Krishna, and J.R. Gillette. 1977. Bioactivation of carbon tetrachloride, chloroform and bromotrichloromethane: Role of cytochrome P-450. Life Sci. 20:1541-1548.
Smith, A.A., P.P. Volpitto, Z.W. Gramling, M.B. DeVore, and A.B. Glassman. 1973. Chloroform, halothane, and regional anesthesia: A comparative study. Anesth. Analg. 52:1-11.
Smith, J.H., and J.B. Hook. 1984. Mechanism of chloroform nephrotoxicity. III. Renal and hepatic microsomal metabolism of chloroform in mice. Toxicol. Appl. Pharmacol. 73:511-524.
Stevens, J.L., and M.W. Anders. 1981. Effect of cysteine, diethyl maleate, and phenobarbital treatments on the hepatotoxicity of [1H]- and [2H] chloroform. Chem. Biol. Interact. 37:207-217.
Stoner, G.D., P.B. Conran, E.A. Greisiger, J. Stober, M. Morgan, and M.A. Periera. 1986. Comparison of two routes of chemical administration on the lung adenoma
response in strain A/J mice . Toxicol. Appl. Pharmacol. 82:19-31.
Sturrock, J. 1977. Lack of mutagenic effect of halothane or chloroform on cultured cells using the 8-azaguanine test system. Br. J. Anaesth. 49:207-210.
Taylor, D.C., D.M. Brown, R. Keeble, and P. F. Langley. 1974. Metabolism of chloroform—II. A sex difference in the metabolism of [14C]-chloroform in mice. Xenobiotica 4:165-174.
Templin, M.V., A.A. Constan, D.C. Wolf, B.A. Wong, and B.E. Butterworth. 1998. Patterns of chloroform-induced regenerative cell proliferation in BDF1 mice correlate with organ specificity and dose-response of tumor formation. Carcinogenesis 19:187-193.
Templin, M.V., K.C. Jamison, C.S. Sprankle, D.C. Wolf, B.A. Wong, and B.E. Butterworth. 1996a. Chloroform-induced cytotoxicity and regenerative cell proliferation in the kidneys and liver of BDF1 mice. Cancer Lett. 108:225-231.
Templin, M.V., K.C. Jamison, D.C. Wolf, K.T. Morgan, and B.E. Butterworth. 1996b. Comparison of chloroform-induced toxicity in the kidneys, liver, and nasal passages of male Osborne-Mendel and F-344 rats. Cancer Lett. 104:71-78.
Templin, M.V., J.L. Larson, B.E. Butterworth, K.C. Jamison, J.R. Leininger, S. Méry, K.T. Morgan, D.C. Wolf, and B.A. Wong. 1996c. A 90-day chloroform inhalation study in F-344 rats: Profile of toxicity and relevance to cancer studies. Fundam. Appl. Toxicol. 32:109-125.
Testai, E., S. DiMarzio, and L. Vittozzi. 1990. Multiple activation of chloroform in hepatic microsomes from uninduced B6C3F1 mice. Toxicol. Appl. Pharmacol. 104:496-503.
Testai, E., F. Gramenzi, S. DiMarzio, and L. Vittozzi. 1987. Oxidative and reductive biotransformation of chloroform in mouse liver microsomes. Mechanisms and models in toxicology. Arch. Toxicol. Suppl. 11:42-44.
Topham, J.C. 1980. Do induced sperm head abnormalities specifically identify mammalian mutagens rather than carcinogens? Mutat. Res. 74:379-387.
Torkelson, T.R., F. Oyen, and V.K. Rowe. 1976. The toxicity of chloroform as determined by single and repeated exposure of laboratory animals . J. Am. Ind. Hyg. Assoc. 37:697-705.
Townsend, E. 1939. Acute yellow atrophy of the liver. Two cases, with one recovery. Br. Med. J. 2:558-560.
Tumasonis, C.F., D.N. McMartin, and B. Bush. 1987. Toxicity of chloroform and bromodichloromethane when administered over a lifetime in rats. J. Environ. Pathol. Toxicol. Oncol. 7:55-64.
Van Dyke, R.A., M.B. Chenoweth, and A.V. Poznak. 1964. Metabolism of volatile anesthetics—I. Conversion in vitro of several anesthetics to 14CO2 and chloride. Biochem. Pharmacol. 13:1239-1247.
Wang, P.-Y., T. Kaneko, A. Sato, M. Charboneau, and G.L. Plaa. 1995. Dose and route dependent alteration of metabolism and toxicity of chloroform in fed and fasting rats. Toxicol. Appl. Pharmacol. 135:119-126.
Whitaker, A.M., and C.S. Jones. 1965. Report of 1500 chloroform anesthetics administered with a precision vaporizer. Anesth. Analg. 44:60-65.
White, A.E., S. Takehisa, E. Shin, I. Edmond II, S. Wolff, and W.C. Stevens. 1979. Sister chromatid exchanges induced by inhaled anesthetics. Anesthesiology 50:426-430.
Wolf, C.R., D. Mansuy, W. Nastainczyk, G. Deutschmann, and V. Ullrich. 1977. The reduction of polyhalogenated methanes by liver microsomal cytochrome P-450. Mol. Pharmacol. 13:698-705.
Wolf, D.C., and B.E. Butterworth. 1997. Risk assessment of inhaled chloroform based on its mode of action. Toxicol. Pathol. 25:49-52.
Yamamoto, S., S. Aiso, N. Ikawa, and T. Matsushima. 1994. Carcinogenesis studies of chloroform in F344 rats and BDF1 mice [abstract]. Proceedings of the Fifty-third Annual Meeting of the Japanese Cancer Association, 2445 Ohshibahara Hirasawa Hando Kanagawa, 257 Japan.