5
Estuaries and Coastal Systems
The northern and southern estuaries of the greater Everglades ecosystem are aquatic habitats that span the transition zones between the inland freshwater riverine and wetland habitats and the marine environments of the Atlantic Ocean, the Florida Keys Reef tract, and the Gulf of Mexico (Figure 5-1). Although they share similar attributes with estuaries around the world, these estuaries are unique within the continental United States due to their subtropical climate, karst geology, and connectivity to the greater Everglades (Table 5-1). South Florida estuaries are beloved by the public and are vital to Florida’s economy—supporting commercial and recreational fisheries, recreation, and tourism. However, as a direct and indirect consequence of an increasing human population, these coastal estuaries are among the most threatened natural habitats in Florida (Scott, 2004). Over the past 150 years they have been impacted by land development and structural changes (e.g., dredge and fill activities), hydromodification, pollution, climate change and sea-level rise, commercial and recreational overuse, and, recently, algal blooms, some of which are toxic.
The extensive die-off of seagrass in Florida Bay during the 1980s and the associated recurring phytoplankton blooms were early motivations for the Central and South Florida Restudy in 1996 and authorization of the Comprehensive Everglades Restoration Plan (CERP). A key CERP goal is to return more natural patterns of flow to the northern estuaries and to send more water south through the remnant Everglades and into the southern estuaries of Florida Bay and Biscayne Bay. Scientists have been monitoring, conducting research, and developing modeling tools that have supported management decisions on performance measures and targets for the northern and southern estuaries to assess the progress of CERP restoration. However, these estuaries are interconnected with and constantly evolving in response to a variety of natural and human-driven stressors. Many of these stressors are outside the direct influence of the CERP and may limit the ability of restoration to contribute to CERP goals. An
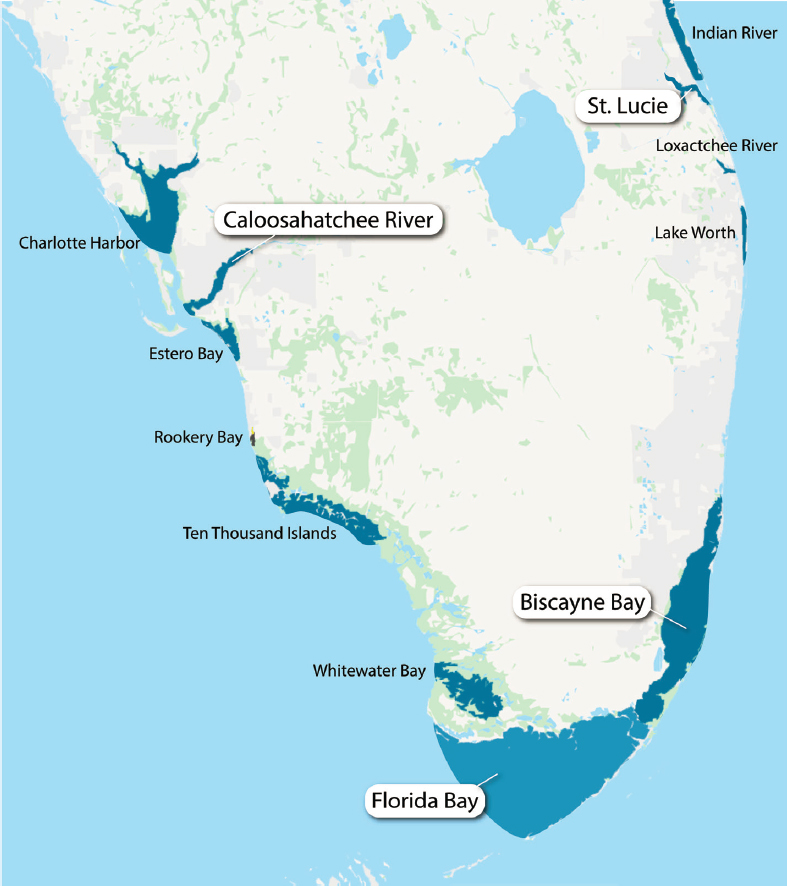
excellent example of an external set of drivers is climate change (see Box 5-1), which is already affecting Florida estuaries (Figure 5-2) and will present a major challenge to the management of water, water quality, and estuarine biological resources of South Florida.
Moreover, CERP projects are coming online among a complex matrix of non-CERP actions and legal prescriptions that can affect both the Everglades and
TABLE 5-1 Comparison of Key Characteristics of Four South Florida Estuaries
Caloosahatchee | St. Lucie | Biscayne Bay | Florida Bay | |
---|---|---|---|---|
Type | River dominated estuary | River dominated estuary | Marine lagoon | Estuarine lagoon |
Estuary size | Area: 65 km2 Length: 42 km | Area: 26 km2 Length: 11 km | Area: 1,100 km2 | Area: 2,200 km2 |
Watershed size | 3,600 km2 | 2,700 km2 | 2,400 km2 | 6,200 km2 |
Estuary size: Watershed size | 0.02 | 0.01 | 0.46 | 0.32 |
Volume (in 106 m3) | 140 | 53 | 2,200 | 2,000 |
Average annual freshwater inflows (in 1,000s AF) | 1,006 | 80 | 1,685 | 331 |
Flushing time (years) | 0.1 | 0.005-0.05 | 1.1 | 6.0 |
N:P in load (molar ratio) | 21-25.2 | 11-19.7 | 274 | 260 |
Trophic state | Mesotrophic | Mesotrophic | Oligotrophic | Oligotrophic |
Limiting nutrient | N | N | P | P, except in far western portions |
Primary focus of CERP restoration | Reduce wet season high flow events; establish dry season optimum flow regime to reduce salinity intrusion | Reduce high and low flow events to optimize salinity regime; reduce loads from watershed via STAs | Improve nearshore salinity and enhance coastal ecosystems; increase flows (in full CERP) | Increase flows to eastern and central bay to lower the frequency and extent of FB hypersalinity events and associated seagrass dieoff |
NOTE: AF, acre-feet; FB, Florida Bay; N, nitrogen; P, phosphorus; STA, stormwater treatment area.
SOURCES: Buzzelli et al., 2013a; Fourqurean, 2019; Glenn, 2019; Graham et al., 2020; Ji et al., 2007.
South Florida estuaries. A broad array of federal and state programs and uses are pursuing sometimes overlapping but often independent purposes and goals that affect the estuaries (Table 3-6). For example, water quality compliance under the Clean Water Act is a state responsibility, although water quality is a critical driver of estuarine conditions and essential to meet CERP goals for seagrass and oysters (see Box 3-1). Within each South Florida estuary, it is presently unclear what role the CERP can play in addressing pressing environmental problems and what additional actions, either through future unplanned CERP projects or through non-CERP efforts, are necessary to mitigate those problems. In this chapter the committee sought to synthesize the following for the northern estuaries (Caloosahatchee River Estuary and the St. Lucie Estuary) and the southern estuaries (Florida Bay and Biscayne Bay):
- Key environmental problems facing each estuary;
- Projections of what CERP projects will do to help resolve these issues, and potential mismatches in stakeholder expectations for the CERP;
- Key questions facing CERP and non-CERP decision makers regarding restoration of the estuaries; and
- Critical science needs (e.g., observations, synthesis, models) to inform these management decisions, which could be addressed in collaboration between CERP and non-CERP agencies.
In the next section on the northern estuaries, these topics are addressed in an integrated format because of the similarities between the estuaries. Then, discussions of these topics follow for Biscayne Bay and Florida Bay.
NORTHERN ESTUARIES
The northern estuaries include the Caloosahatchee River Estuary, Estero Bay, and southern Charlotte Harbor on the west coast and the St. Lucie Estuary, southern Indian River Lagoon, Lake Worth Lagoon, and Loxahatchee River Estuary on the east coast (Figure 5-1). These estuaries are hotspots of biological
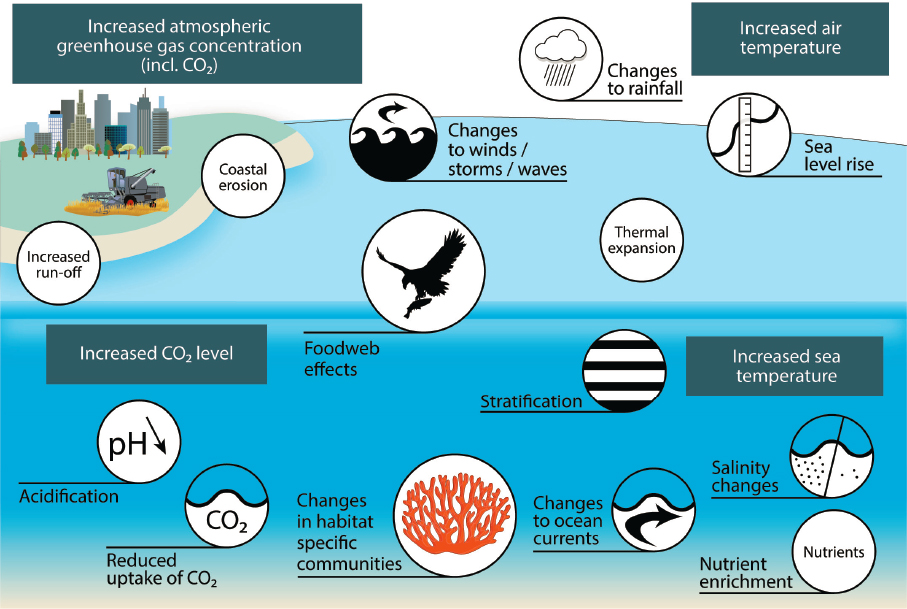
SOURCE: Adapted from OSPAR, 2010.
diversity and also fuel productive South Florida coastal economies; for example, the St. Lucie Estuary and Indian River Lagoon supported an estimated $7.6 billion in total annual direct economic output from activities such as tourism, fisheries, and marine industries (East Central Florida and Treasure Coast Regional Planning Council, 2016). Among these seven northern estuaries, the Caloosahatchee River and St. Lucie estuaries are the most directly impacted by Lake Okeechobee water releases and numerous CERP projects. In this section, the committee describes environmental changes to the Caloosahatchee River and St. Lucie estuaries and near-term CERP progress toward restoration goals, identifies key questions in northern estuaries restoration management, and reviews the adequacy of science to support these decisions.
Environmental Changes and Their Ecological Effects
Structural and hydrologic changes in the Caloosahatchee River and St. Lucie estuaries and watersheds have resulted in substantial water quality changes and ecological impacts in the estuaries.
Caloosahatchee River Estuary
The Caloosahatchee River Estuary is a river-dominated estuary some 42 km in length (Figure 5-3). Its watershed encompasses approximately 3,600 km2 (1,400 mi2). Under predrainage conditions, no navigable connection existed between the Caloosahatchee River and Lake Okeechobee, although during rainy periods, some Lake Okeechobee water overflowed into the headwaters
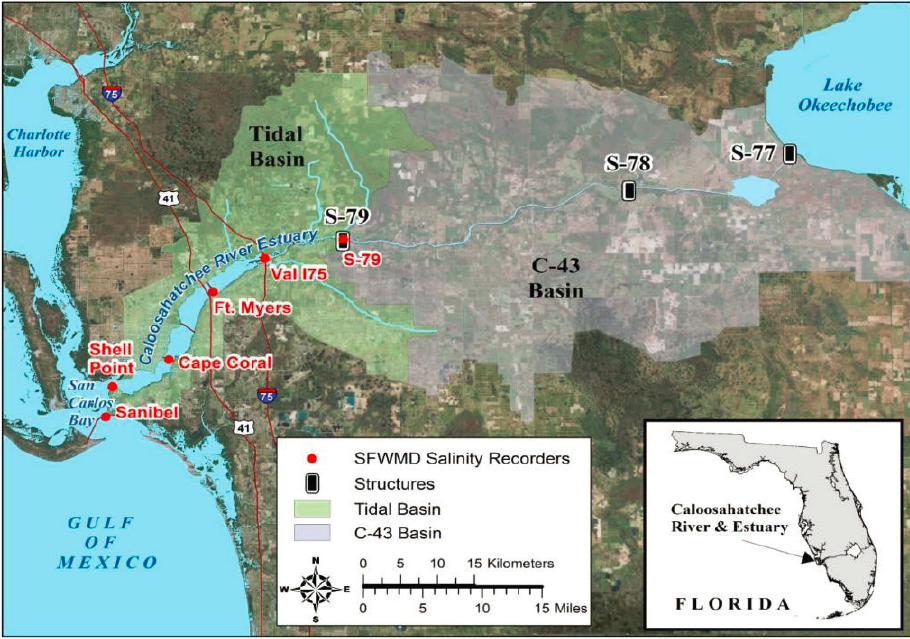
SOURCE: Glenn, 2019.
of the Caloosahatchee River (Steinman et al., 2002). More than 150 years ago, the Caloosahatchee River Estuary was home to abundant beds of brackish water seagrass (Vallisneria americana) in the low-salinity zones in the upper estuary and marine seagrasses (Thalassia testudinum, Halodule wrightii) near the estuary mouth (Figure 5-3). These beds retained sediments, attenuated wave action, improved water clarity and quality, and hosted an abundance of aquatic life including fish, shellfish, aquatic mammals, freshwater turtles, and birds (CHNEP, 2016; FWC, 2016; FWS, 2016b). Under predrainage conditions, extensive oyster bars and shoals covered the lower estuary (Sackett, 1888). A rich spawning and nursery habitat for invertebrates and finfish supported economically important recreational and commercial fisheries, contributing to Ft. Myers’ fame as a tourism and sportfishing magnet (Kokomoor, 2012). The estuary continues to provide forage and breeding grounds for resident and migratory birds and marine mammals; more than 40 state and federally threatened and endangered species utilize the Caloosahatchee River Estuary as critical habitat, including the iconic West Indian manatee (CHNEP, 2016).
Structural and hydrologic changes.
Many of the ecological problems in the Caloosahatchee River Estuary are a result of high variability in seasonal and interannual freshwater discharge and the structural changes within the estuaries and their respective watersheds that took place over the past century. In the Caloosahatchee River watershed, riverine hydrology was radically altered, starting in the 1880s when the river was deepened and straightened (Antonini et al., 2002). By 1918, a combination of locks and spillways was added to the river, from the Franklin Lock and Dam (S-79), which now forms the upstream head of the estuary, to the S-77 outflow structure, which connected the headwaters of the Caloosahatchee River to Lake Okeechobee (Figure 5-3). This hydrologic connection to Lake Okeechobee expanded the estuary’s watershed1 and increased the occurrence of high-volume flows. Over the past two decades, on average, contributions from the local watershed represented the majority of the inflows to the estuary (Figure 5-4), but Lake Okeechobee regulatory releases have contributed up to 43 percent of total flows in recent wet years (see Figure 3-21; Serna et al., 2020). In the 1960s navigation channels were dredged, creating a conduit for migration of saltwater up the estuary, and historic oyster bars were mined for road construction (Chamberlain and Doering, 1998; SFWMD, 2018a; Sun et al., 2016). River channelization, wetland drainage, and urban and agricultural development reduced natural water storage throughout the watershed and altered the amount and timing of freshwater flow into the estuary. These changes dramatically altered mesohaline and polyhaline habitats of the mid and
___________________
1 The SFWMD does not include Lake Okeechobee or the Kissimmee River Basin as part of the delineated area of the estuary watershed.
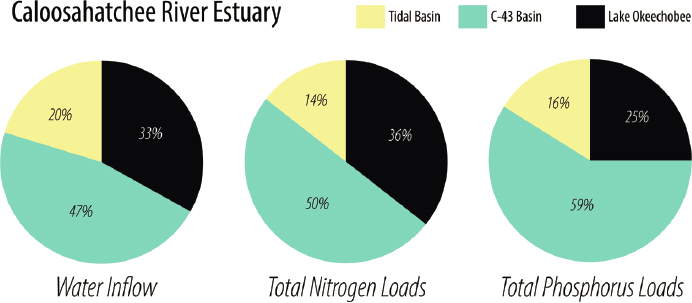
SOURCE: Data from Serna et al., 2020.
lower estuary, which have important consequences for its ecology and water quality (Graham et al., 2020).
Ecological and water quality implications.
These structural and hydrologic changes have modified salinity regimes and caused decline in water quality in ways that fundamentally altered suitable habitat for brackish water and marine seagrass, oysters, plankton, and fish in the Caloosahatchee River Estuary (Barnes, 2005; Chamberlain and Doering, 1998). Extended high wet season discharges to the Caloosahatchee River Estuary reduce salinities, causing mortality of oysters and marine seagrasses in the lower estuary, while associated high colored dissolved organic matter (CDOM)2 throughout the estuary limit light penetration, inhibiting Vallniseria growth (Chamberlain and Doering, 1998; Doering et al., 2002; Volety et al. 2009). Areas that were dredged and deepened would preclude seagrass, particularly when coupled with lower light availability. Harris et al. (1983) estimate a loss of 87 percent of marine seagrass from the lower estuary. Extremely low salinities from these discharges are also thought to be responsible for the presence of the fungus Aphanomyces invadens and the occurrence of fish with lesions in the Caloosahatchee River Estuary (Sosa et al., 2007).
Conversely, low dry season freshwater discharges and associated high salinity in the upper estuary caused die-back of Vallniseria beds. Periods of
___________________
2 Although CDOM is comprised of naturally occurring organic matter, developed land uses including draining and clearing of wetlands and adjacent riparian habitat can greatly augment CDOM.
low flow in the 2000s resulted in the loss of the majority of Vallniseria acreage (Figure 5-5), and even when salinity conditions have been within tolerance ranges, revegetation has been hampered by reduced dispersal (Graham et al., 2020). In the dry season without sufficient freshwater inflows, saltwater migrates all the way up the estuary to the S-79 structure, truncating the salinity gradient and compressing estuarine habitat for estuarine plankton and fish (SFWMD, 2017), increasing predation and competition for food resources, and lowering growth and survival (Eby and Crowder, 2002; Peterson, 2003; SFWMD, 2018a). Low flows and salinity intrusion into the upper estuary enhance stratification, which exacerbates the potential for algal blooms and low dissolved oxygen. Oyster production has generally been low, because the life cycle is impacted by both low and high salinities (i.e., < 10 or > 30 practical salinity units [PSU]; SFWMD, 2020; Volety et al., 2009).
Structural, hydrologic, and land use alterations in the watershed and estuary have promoted water quality conditions that have caused low dissolved oxygen in some local tidal basins; reduced water clarity, which diminishes aesthetics and light required for seagrass growth; and, more recently, supported recurring toxic harmful algal blooms (HABs; see Box 5-2). In 2009, a total maximum daily load (TMDL) was established to limit nitrogen loading from the Caloosahatchee River watershed associated with low dissolved oxygen (see Chapter 3 for discussions of
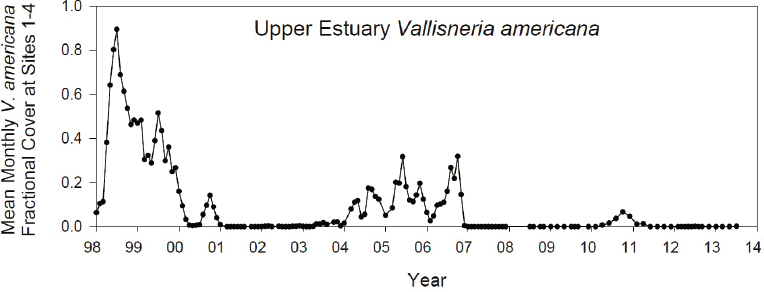
SOURCE: RECOVER, 2014.
recent water quality relative to the TMDL), but the TMDL did not address water clarity or HABs, which have more recently emerged as key environmental problems. CDOM is the most important factor limiting water clarity, and increased freshwater flows to the estuary are associated with increases in CDOM (Figure 5-6; Buzzelli et al., 2014a; Chen et al., 2015). Increased flows and nutrient loads and water column stratification are among the factors known to promote freshwater cyanobacterial blooms (Paerl and Otten, 2013) and marine HABs such as Karenia brevis (Medina et al., 2020). Although the Caloosahatchee watershed is the largest source of nutrient inputs (Figure 5-4; see also Chapter 3), discharges from Lake Okeechobee are an additional source of nutrients and may also seed populations of cyanobacteria (Phlips et al., 2012). Climate change exacerbates factors that lead to increased HAB frequency, such as warm temperatures, high irradiance, and high carbon dioxide (Burford et al., 2020; Hallegraeff, 2010). As climate change shortens the duration of the cooler seasons, the frequency of HABs in inland and coastal waters is expected to increase (see Box 5-1; Chapra et al., 2017).
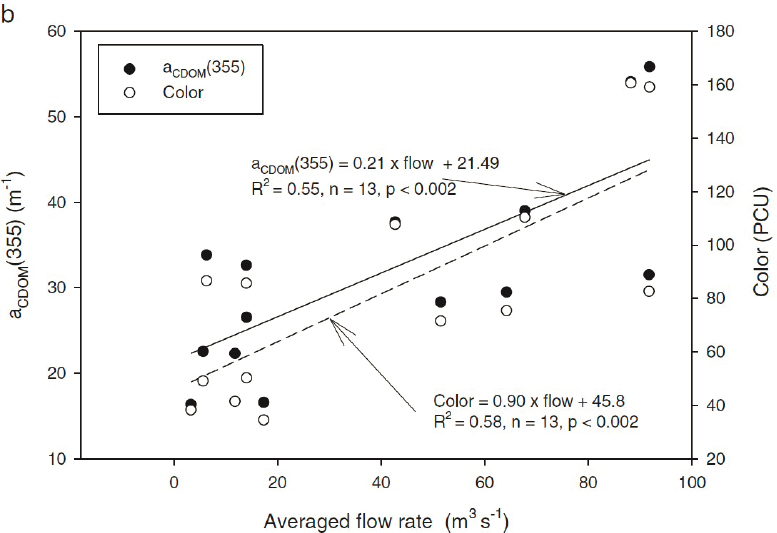
SOURCE: Chen et al., 2015.
St. Lucie Estuary
To the east of Lake Okeechobee, the St. Lucie Estuary is a river-dominated estuary, extending 11 km long to its outlet to the southern Indian River Lagoon, through which it connects to the Atlantic Ocean (Figure 5-7). However, in the mid- to late 1800s, the St. Lucie was a freshwater lagoon, embedded in a mosaic of freshwater wetlands and upland prairie with an ephemeral connection to the Indian River Lagoon (SFWMD, 2002). In 1892, an inlet was permanently dredged, converting the freshwater lagoon to a brackish water estuary (Osborne, 2016; SFWMD, 2002). Like the Caloosahatchee, 100 years ago the St. Lucie Estuary and the nearby southern Indian River Lagoon were home to abundant marine and brackish-water seagrass meadows and oyster beds. This estuary and its adjacent marine lagoon fostered a rich and biologically diverse flora and
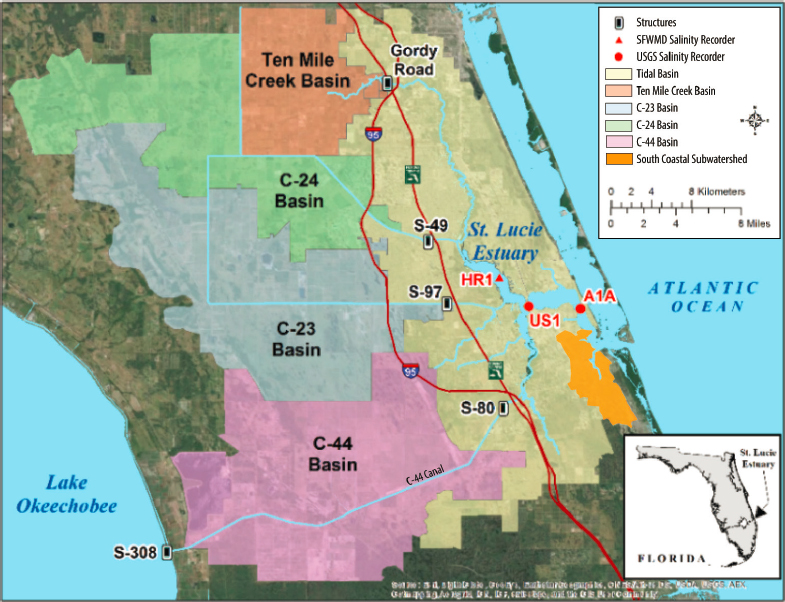
SOURCE: Modified from Glenn, 2019.
fauna including more than 2,000 species of plants, 600 species of fish, and 300 species of resident and migratory birds, including 53 threatened or endangered species (Osborne, 2016). During the early 20th century, the St. Lucie–Indian River Lagoon estuarine complex was renowned for its recreational and commercial fisheries, including clams, crabs, oysters, shrimp, and finfish (e.g., inshore tarpon). These fisheries were key elements that attracted tourism and urban development, leading to the nickname, “The Treasure Coast” (Osborne, 2016).
Structural and hydrologic changes.
Like the Caloosahatchee River Estuary, the St. Lucie Estuary has a long history of structural alterations and water quality changes, with parallel yet distinct biological consequences. In 1916, the C-44 Canal was constructed, connecting Lake Okeechobee to the South Fork of the St. Lucie (Figure 5-7). With the accompanying boom in agriculture and urban development, the natural watershed was drained and interconnected, expanding the St. Lucie watershed area three-fold (SFWMD, 2002; Sime, 2005). The expanded drainage area created a watershed-to-estuarine area ratio that is 100 to 1,000 times higher than is typical of estuarine environments around the world—an extreme outlier (Table 5-1; Dürr et al., 2011). The canal drainage system greatly reduced natural water storage in the St. Lucie watershed, exacerbating floods and drought conditions in the estuary. Operation of the Lake Okeechobee regulatory releases for flood control combined with the basin drainage system significantly altered the magnitude and timing of freshwater flow into the St. Lucie, with the lake contributing about 26 percent of the total inflow (Figure 5-8), whereas historically the estuary was not connected to the lake.
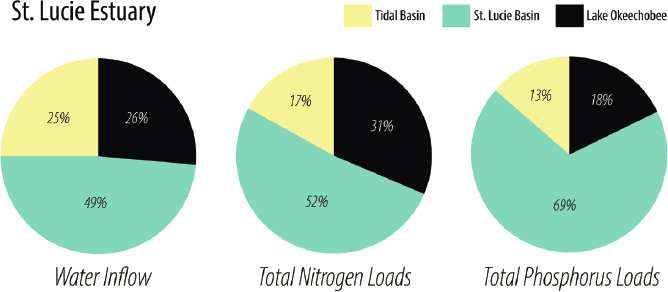
SOURCE: Adapted from Serna et al., 2020.
Natural shorelines of the St. Lucie Estuary were also developed and hardscaped to prevent erosion from boat traffic.
Water quality and ecological impacts.
As with the Caloosahatchee River Estuary, wet season watershed runoff and Lake Okeechobee regulatory releases bring extreme fluctuations in St. Lucie Estuary salinity and plumes of associated suspended sediment, nutrients, and CDOM (Chen et al., 2015; Sime, 2005). CDOM and turbidity are a major water quality issue, because they limit light to the estuary bottom. Together with highly variable salinity, these water quality changes have caused a tipping point, converting the estuary from a seagrass-dominated habitat to a plankton-dominated ecosystem (Graham et al., 2020; Sime, 2005). The composition of the phytoplankton community is strongly controlled by freshwater discharges and associated nutrients from the St. Lucie Basin and Lake Okeechobee and is intermittently dominated by toxic HABs (see Box 5-2; Badylak et al., 2015). Local watershed inputs from the St. Lucie Basin are a more significant source of nutrient loading and CDOM than Lake Okeechobee (Figure 5-8; Walsh, 2017). The Florida Department of Environmental Protection (FDEP) established a TMDL for nitrogen and phosphorus loading for the St. Lucie watershed to achieve nutrient and dissolved oxygen criteria in the estuary (FDEP, 2008), but the TMDL was not specifically intended to address HABs.
High CDOM and sediment plumes from freshwater discharges limit seagrass extent as far seaward as the southern Indian River Lagoon (Buzzelli et al., 2012). High sedimentation events from the watershed cause muck deposits in the estuary, resulting in unsuitable substrates for oysters, benthic invertebrates, and seagrass. These sediments have a high oxygen demand, creating occasional hypoxia events in the estuary (Buzzelli and Doering, 2019; FDEP, 2008), and contain concentrations of trace metals that are potentially harmful to fish and invertebrates (Sime, 2005). Collectively, by the mid-20th century, these habitat changes led to a collapse of the historic and world-renowned inshore tarpon fishery that relied on the native food web (Osborne, 2016). Over the past several decades, these conditions, particularly low-salinity events, have caused a loss of approximately 85 percent of native oyster habitat, from a historical extent of 567 hectares (1,400 acres; Sime, 2005). Current oyster production in the remaining habitat shuts down during low-salinity events.
Restoration Goals and Expected Effects of Planned CERP Projects
The 2007 Interim Goals Agreement (USACE et al., 2007) describes the early qualitative CERP hydrologic and ecological goals for the Caloosahatchee River and St. Lucie estuaries. These CERP goals aimed to increase American oyster and seagrass habitat by managing flow regimes to improve salinities for
those habitats. Specifically, the Interim Goals aimed to reduce the occurrence and duration of high-volume flows (> 2,800 cubic feet per second [cfs] in the Caloosahatchee and > 2,000 cfs in the St. Lucie) as well as low-volume flows (< 450 cfs in the Caloosahatchee and < 350 cfs in the St. Lucie), measured as monthly averages. To track restoration progress and understand factors underlying observed changes, RECOVER monitors oysters (density, reproduction and recruitment, disease) and seagrass (species occurrence, cover, density) as well as other biological and water quality indicators including salinity and water clarity (CDOM, turbidity) (RECOVER, 2019). CERP planners did not explicitly consider water quality or HABs when establishing these 2007 goals.
Several CERP projects (USACE and SFWMD, 1999) improve the ability to manage the magnitude and timing of freshwater flow to the northern and southern estuaries, and most of these have now been planned (Table 5-2; see also Chapter 3 for project descriptions and progress to date). These planned projects enhance water storage to the north, south, east, and west of Lake Okeechobee, including large aboveground reservoirs and belowground storage using aquifer storage and recovery (ASR). Collectively, these projects include about 676,000 acre-feet (AF) of aboveground storage capacity and 80 ASR wells with the potential for each to inject or withdraw 5 million gallons per day into the subsurface. The potential for additional storage in the development of the new Lake Okeechobee System Operating Manual remains the largest unresolved element affecting the northern estuaries (see Chapter 3).
TABLE 5-2 Planned CERP Projects Affecting the Northern Estuaries
Storage Component | Storage Capacity (acre-feet) | Maximum Annual Storage Capacity (acre-feet/year) |
---|---|---|
Aboveground Reservoirs | ||
Lake Okeechobee Watershed Restoration Plan | 46,000a | |
C-43 Reservoir | 170,000 | |
Indian River Lagoon-South | 160,000b | |
Central Everglades Planning Project (CEPP, includes EAA Reservoir) | 300,000c | |
ASR Wells | ||
Lake Okeechobee Watershed Restoration Plan | 448,000a |
a Based on tentatively selected plan for the Lake Okeechobee Watershed Restoration Project as of January 2020.
b Includes C-44, C-23, C-24, C-25, and St. Lucie North and South Fork reservoirs and natural storage areas.
c Includes Everglades Agricultural Area (EAA) Reservoir and A-1 flow equalization basin, which was constructed for Restoration Strategies.
SOURCES: NRC, 2005; USACE and SFWMD, 1999, 2004b, 2010, 2014.
CERP Projects That Affect the Caloosahatchee River Estuary
The three primary CERP projects that are expected to improve conditions in the Caloosahatchee River Estuary are the C-43 Reservoir, the Central Everglades Planning Project (CEPP; with Everglades Agricultural Area [EAA] Reservoir), and the Lake Okeechobee Watershed Restoration Project. As of January 2021, of these three, only the Lake Okeechobee Watershed Restoration Project has not yet been authorized. Collectively, the projects are predicted to reduce the number of high-volume mean monthly flows (>2,800 cfs) to the Caloosahatchee River Estuary by 43 percent and very-high-volume mean monthly flows (>4,500 cfs) by 72 percent. These projects collectively meet the performance originally predicted for the CERP (termed here as the CERP goal; see Table 5-3), which emphasized reduction of high flows from Lake Okeechobee over basin flows.
Planned and approved CERP projects will have a larger effect on low-flow events in the Caloosahatchee River Estuary. CERP projects are projected to reduce the number of mean monthly flows below 450 cfs by about 70 percent, although further performance improvements may be feasible with changes to Lake Okeechobee operations (W. Wilcox, South Florida Water Management District [SFWMD], personal communication, 2020). If future changes in Lake Okeechobee operations (see Chapter 3) reduce the availability of freshwater to the estuaries during dry periods, the predicted project performance could be reduced. The stated ecosystem restoration target is zero low-flow events (Table 5-3).
CERP Projects That Affect the St. Lucie Estuary
As in the Caloosahatchee, the central objective of the CERP for the St. Lucie Estuary is to reduce the occurrence and duration of harmful high- and low-volume discharges. Three CERP projects are expected to have significant effects on the St. Lucie: the Indian River Lagoon-South Project, the CEPP (with EAA Reservoir), and the Lake Okeechobee Watershed Restoration Project (Table 5-2). Results of model simulations show that these projects are expected to reduce the overall number of 14-day periods with high flows in the St. Lucie Estuary (14-day moving averages over 2,000 cfs) by 66 percent and the number of high-flow periods resulting from Lake Okeechobee regulatory releases by 82 percent (Table 5-4). The projects collectively are predicted to be more successful at reducing the number of high-flow events from Lake Okeechobee compared to those from local basin runoff (55 percent reduction).
The planned and approved CERP projects appear less successful at reducing the frequency of low-flow conditions in the St. Lucie Estuary. Collectively, the CERP projects in Table 5-2 provide a 20 percent reduction in the number
TABLE 5-3 Predicted Effects of CERP Projects on the Number of Months with High and Low Flows in the Caloosahatchee Estuary Based on a 41-Year Period of Record
Number of Months with High-Volume Mean Monthly Flows (>2,800 cfs) | Number of Months with Very-High-Volume Mean Monthly Flows (>4,500 cfs) | Number of Months with Low Mean Monthly Flows (<450 cfs) | |
---|---|---|---|
Existing conditions baseline (2014) | 94a | 43b | 116a |
C-43 | 81a | 33b | 27a |
C-43+CEPP | 70a,c | 29c | 23a |
C-43+CEPP PACR | 61c | 24c | 26d |
C-43+CEPP+LOWRP | 60e | 25e | 24e |
C-43+CEPP PACR+LOWRP | 54f | 12f | 37f,g |
CERP goal | 81% reduction in lake-triggered events (56 total)h | ||
Restoration target | 0d | 0d | 0d |
NOTES: Output from several different model runs based on 1965-2005 precipitation data. Output reflects on the number of months meeting criteria out of the 41-year (or 492-month) period of record used in the analyses. The model runs presented here may not have the exact same conditions from project to project, but the output collectively is presented for general trends. CEPP, Central Everglades Planning Project; LOWRP, Lake Okeechobee Watershed Restoration Project; PACR, Post Authorization Change Report. SOURCES:
a USACE and SFWMD (2014, Figure G-28).
b USACE and SFWMD (2014, Figure 6-7).
e USACE and SFWMD (2019, Chap. 6).
f W. Wilcox, SFWMD, personal communication, 2020.
g “This ‘reversal’ in low flow performance here can be overcome with Lake [Okeechobee] operations – see CERP Component E5.” (W. Wilcox, SFWMD, personal communication, 2020).
h The CERP goal, as defined by the RECOVER CERPA model run compared to existing-conditions baseline (ECB), using 1965-2000 data, is an 81 percent reduction in lake-triggered events. Using 1965-2005 data, this represents 56 months of high-volume flows, with approximately 47 months triggered by local basin flows (W. Wilcox, SFWMD, personal communication, 2020).
of months with flows < 350 cfs compared to an ecosystem restoration target of 66 percent reduction (Table 5-4).
Finally, the Indian River Lagoon-South project includes 8,700 acres of stormwater treatment areas (STAs), which is expected to reduce nutrient loads to the St. Lucie Estuary. Though these projections are dated, the U.S. Army Corps of Engineers (USACE) and SFWMD (2004b) state that the STAs would reduce phosphorus loads by 18 percent and nitrogen loads by up to 8 percent compared to the 2050 conditions without the project. Considering all aspects of the project, including nutrient removal due to natural land restoration, increased irrigation,
TABLE 5-4 Predicted Effects of CERP Projects on the Number of 14-Day Periods or Months with High and Low Flows in the St. Lucie Estuary Based on a 41-Year Period of Record
Number of High-Volume 14-Day Moving Average Flows (>2,000 cfs) | Local Basins Only: Number of High-Volume 14-Day Moving Average Flows (>2,000 cfs) | Lake O. Reg. Releases Only: Number of High-Volume 14-Day Moving Average Flows (>2,000 cfs) | Number of Months with Low Mean Monthly Flows (<350 cfs) | |
---|---|---|---|---|
Existing-conditions baseline (2014) | 177a | 105a | 72a | 89a |
IRL-S | 151a | 86a | 65a | 92a |
IRL+CEPP | 86a | 50a | 36a | 65a or 83b |
IRL+CEPP PACR | 71c | 49c | 22c | 67c |
IRL+CEPP+LOWRP | 71b | 52b | 19b | 83b |
IRL+CEPP PACR+LOWRP | 60d | 47d | 13d | 71d |
CERP goal | 81% reduction in eventse | |||
Restoration target | 0a | 31f |
NOTES: Output from several different model runs based on 1965-2005 precipitation data. These models may not use the exact same assumptions, but the output collectively is presented for general trends. CEPP, Central Everglades Planning Project; IRL-S, Indian River Lagoon-South; LOWRP, Lake Okeechobee Watershed Restoration Project; PACR, Post Authorization Change Report.
SOURCES:
a USACE and SFWMD (2014, Figure G-29).
b USACE and SFWMD (2019, Chap. 6).
d W. Wilcox, SFWMD, personal communication, 2020.
e The CERP goal, as defined by the RECOVER CERPA model run compared to existing conditions baseline, using data from 1965 to 2000, is an 81% reduction in lake-triggered events (W. Wilcox, SFWMD, personal communication, 2020).
and passive removal in the reservoir, the project was expected to reduce phosphorus loads to the St. Lucie Estuary by 35 percent and nitrogen loads by 24 percent compared to the 2050 conditions without the project.
Overall Effects from Planned CERP Projects on the Northern Estuaries
As noted previously, the majority of flows and nutrient loads to the Caloosahatchee River and St. Lucie estuaries drain from local basins, with the remainder from Lake Okeechobee (Figures 5-4 and 5-8). Thus, while planned CERP projects are projected to reduce the number of Lake Okeechobee–triggered high-flow events by 80 percent, the overall reduction in the number
of high-volume mean monthly flows is projected to be only 43 percent for the Caloosahatchee River Estuary and 66 percent for the St. Lucie Estuary. Planned CERP projects are predicted to reduce the number of mean monthly low-volume flows for the Caloosahatchee River and St. Lucie estuaries by 70 and 20 percent, respectively.3 Thus, both the St. Lucie and Caloosahatchee are expected to continue to receive damaging high-volume discharges, primarily from their local watersheds, and face low-flow conditions with planned CERP projects. Based on estimated storage needs from Graham et al. (2015), an additional 230,000 AF of storage in the Caloosahatchee River watershed, 40,000 AF in the St. Lucie Basin, and at least 200,000 AF north and/or south of Lake Okeechobee4 beyond currently planned projects would be needed to reduce high flows to the northern estuaries. Additionally, with the exception of the STAs in the Indian River Lagoon-South Project, the CERP will not address nutrient concentrations in the remaining flows, although overall nutrient loads from Lake Okeechobee are expected to be reduced. The shortage in storage to address the local runoff from highly altered watersheds may prevent CERP from reaching its ecological restoration goals.
Implications of Environmental Issues for CERP and Non-CERP Efforts
In the next several years, new CERP storage projects with the potential to improve conditions in the northern estuaries will come online along with the completion of the Herbert Hoover Dike around Lake Okeechobee. Additional CERP and non-CERP projects are also planned in the estuary watersheds over the next decade. The benefits of these projects will depend on their optimized operations, potentially affecting water supply, water quality, HABs, fisheries, aesthetics, and ecotourism, among others.
The CERP and agencies working to restore the northern estuaries have a fundamental challenge: the public is demanding immediate action to eliminate HABs, while also expecting that the CERP will restore seagrass and oyster habitat, while balancing water resource needs and flood risk management for a thriving South Florida economy (Figure 5-9; Graham et al. 2015). Currently, major decisions on the operations of Lake Okeechobee and its water releases
___________________
3 Expected outcomes would change with system operations (including management of Lake Okeechobee) that differ from those modeled.
4Graham et al. (2015) estimated that a total of 400,000 AF of storage in the Caloosahatchee River watershed, 200,000 AF in the St. Lucie River watershed, and approximately 1 million AF of surface storage north and south of Lake Okeechobee would be needed to reduce damaging estuary discharges. Estimates of storage gaps are calculated based on totals in Table 5-2, with an understanding that maximum annual subsurface storage volumes may not equate to surface storage volumes in terms of benefits.
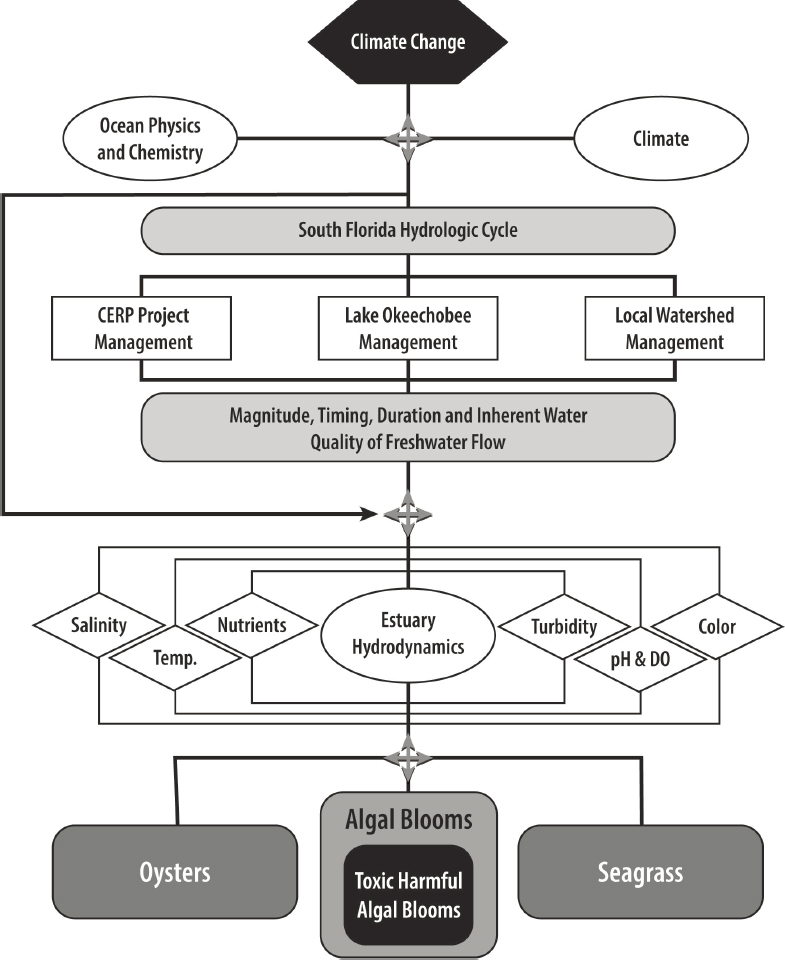
NOTE: DO, dissolved oxygen.
are made without a quantitative understanding of the effects of those decisions on water quality and harmful algal blooms. Additionally, the approaches used by the CERP to estimate optimal freshwater flows for oyster and seagrass habitat restoration (RECOVER, 2020a) primarily rely on salinity tolerance ranges and are lacking additional fundamental habitat and water quality constraints that will ultimately limit their distribution (e.g., sediment quality, nutrients, algal blooms, turbidity, water color).
On the flip side, water quality is inextricably linked to flow, so water quality managers must understand how water releases associated with CERP projects will impact water quality and attainment of TMDLs. For example, nutrient TMDL targets established for both the Caloosahatchee River and St. Lucie estuaries do not consider the ecological consequences of CERP-controlled flows causing a seasonal shifting of nutrient loading toward larger loads during the dry season and smaller loads during the wet season, factors that could influence HABs.
Managers cannot begin to collectively understand system trade-offs and weigh difficult policy choices without a quantitative understanding of the interconnectivity of these environmental drivers and ecosystem problems and their linkage to regional water management (Box 5-3). Additionally, if the role of these different drivers is not better understood, CERP management may be criticized for adverse outcomes—for example, not meeting its goals for seagrass and oyster habitat restoration or harmful algal blooms—although these may be caused primarily by factors beyond the reach of the CERP.
Key Questions for Decision Makers on Restoration of the Northern Estuaries
All parts of the South Florida ecosystem—including the human and natural landscape—share a common water resource within a strongly interconnected hydrologic system. Human and natural ecosystem needs are synchronous and competing, exacerbated by the natural wet and dry cycles of Florida climate, the variability of which is further heightened by climate change (Graham et al., 2015). Therefore, water management decisions inevitably involve trade-offs and difficult policy decisions. Managers will need science to address critical questions such as those posed in Box 5-4 to better inform these decisions.
Science Support for Restoration Management Decisions in the Northern Estuaries
Meeting the challenge of restoring the northern estuaries will require an advanced set of decision support tools—monitoring, research, syntheses, and modeling—in combination with effective coordination and communication
among scientists and water quality and natural resource managers to collectively develop and implement these tools to support decision making. As the CERP moves from restoration planning to project execution, tools will be needed that can evaluate trade-offs in system operation between HABs, water quality, seagrass and oyster restoration, and water supply under current and future conditions, considering system interconnectivity and nonlinear feedbacks. Such tools could be used to quantify understanding of management alternatives; they would support long-term regional planning and coordinated management actions among CERP, water quality, and natural resources managers. In this section, the status of science and readiness of observations, synthesis, and statistical and numerical modeling tools to meet this challenge are reviewed and key priorities for their incremental development are identified.
Integrated Hydrologic System Observations and Modeling
Basin-scale, watershed and estuarine hydrology observations and models have been well synchronized and validated for the northern estuaries over the past two decades. Models consist of (1) regional-scale “basin” models that predict flows based on current or natural system conditions using a hindcast of precipitation and climate data (e.g., 1965-2005), (2) finer-scale models of watershed (pollution) loading for water quality analyses or hydrologic models that optimize design and operations at the project scale, and (3) estuarine hydrodynamic models (Figure 5-10 and Table 5-5). Capabilities of these models to predict the influence of water management and land use on watershed freshwater flows to northern estuaries is generally high. A common set of estuarine hydrodynamic models has been validated against observations for water, salinity, and heat budgets (Ehlinger et al., 2019; FDEP, 2008; Ji et al., 2007; Qiu and Wan, 2013; SFWMD, 2018d; Tetra Tech, 2017) and used to assess water quality (FDEP, 2008; Tetra Tech, 2017) and minimum flow requirements (SFWMD, 2002, 2018d). Hydrologic basin models and estuarine hydrodynamic models have been a scientific workhorse for the CERP. They are used to predict the salinity ranges as a function of ocean forcing and prescribed freshwater flows, informing discussions on high-volume and low-flow targets needed to meet salinity performance measures (Ehlinger et al., 2019). Overall, the readiness of the integrated hydrologic system toolkit to serve as decision support for management of water flows into the northern estuaries is high and the explicit scientific needs in the short term are low.
Use of existing hydrologic models to predict the effects of climate change is a near-term high priority, considering the past and potential future effects of climate change on the South Florida ecosystem (NASEM, 2016; NRC, 2014; SFWMD, 2009), and efforts have been relatively limited to date. The SFWMD published
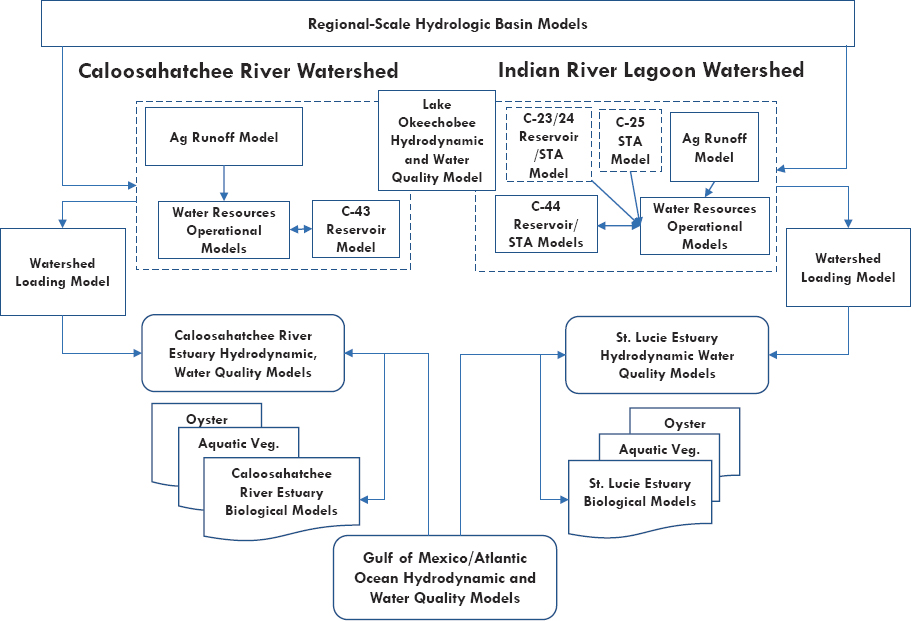
two reviews of the impacts of climate warming, drought, and sea-level rise on the hydrologic cycle and South Florida infrastructure (SFWMD, 2009, 2011), and researchers have conducted limited scenario analyses on the impacts of climate change and sea-level rise on the ecosystem (e.g., Flower et al., 2017; Koch et al., 2015; Obeysekera et al., 2015). NASEM (2016, 2018) outlined a broader approach to examine the implications of possible future climate scenarios, including changes in precipitation (e.g., average, seasonality, extremes), temperature, and sea-level rise on the South Florida ecosystem. A similar strategy was recommended by Graham et al. (2020), but such modeling analyses have not been conducted by CERP agencies. The SFWMD hydrologic models in current use are capable of predicting outcomes based on a range of potential precipitation and climate scenarios constructed from downscaled climate predictions.
TABLE 5-5 Examples of Regional-Scale, Watershed and Estuary Hydrodynamic and Water Quality Models Applied to the Caloosahatchee River and St. Lucie Watersheds
Name | Description |
---|---|
Regional-scale hydrologic and water resources management models | |
Regional Simulation Model (RSM) and Natural System RSM (NSRSM) | The RSM simulates surface- and groundwater hydrology for current or future (RSM) or predrainage (NSRSM) conditions in response to historic climate records and data. The model outputs water levels and flows from canals, water control structures, local topography, and storage reservoirs, etc. It simulates the movement and distribution of water in conjunction with the coordinated operation of canals and structures (Bras et al., 2019; SFWMD, 2005). |
South Florida Water Management Model (SFWMM) | Regional-scale operational model that simulates the major components of the hydrologic cycle in South Florida on a daily basis and analyzes operational changes to the water management system in South Florida (SFWMD, 2005). |
Reservoir Optimization Model | Optimizes reservoir operations to meet the estuarine flow requirements and supplemental irrigation needs and provides day-to-day operational support for reservoirs and STAs in the watershed. Most recent version (OPTI7) used to determine optimal operating rules for detention reservoirs in the northern estuaries (Labadie, 2004). |
Hydrodynamic and water quality models (lake, watershed, and estuary) | |
Lake Okeechobee Water Quality Model | Simulates Lake Okeechobee water budget, temperature, CBOD, dissolved inorganic and organic nitrogen, phosphorus, phytoplankton biomass, cyanobacterial biomass, TSS (James, 2016) |
Watershed Model (WaSH) | Hydrologic model; components are comprised of surface-water flow, groundwater flow, channel flow, and water management practices (SFWMD, 2018d). Used in the CRE and STL; regions with high groundwater and dense array of drainage canals; this model is capable of simulating hydrology for such regions (SFWMD, 2018a). |
Hydrological Simulation Program Fortran (HSPF) | Simulates CRE watershed DO, BOD, temperature, TSS, sediment, ammonia (NH3), nitrite-nitrate (NOx), organic nitrogen (OrgN), orthophosphate (PO4), organic phosphorus (OrgP), and phytoplankton (Tetra Tech, 2017). |
Curvilinear Hydrodynamics in 3D (CH3D) | Time-varying 3D numerical hydrodynamic model used in both the CRE and STL. Coupling of the model with other modules (sediment transport and water quality) makes CH3D an integrated modeling system capable of simulating water quality and other estuarine processes (Sun et al., 2016; Wan et al., 2012). |
Environmental Fluid Dynamics Code (EFDC) | An EPA-sponsored 3D hydrodynamic and water quality model that transports salinity, temperature, simple constituents (e.g., tracer), sediments, and toxic contaminants (e.g., metals or organics; Tetra Tech, 2017). |
Ecological models | |
Oyster, Habitat Suitability Index | Calculates habitat suitability for larval and adult oysters as a function of salinity, temperature, flow for larvae, and substrate for adults in the CRE (Barnes et al., 2007). |
Oyster, Mechanistic | Predicts adult oyster survival related to freshwater inflows and salinity in the CRE. Model incorporates filtration rate, assimilarion efficiency, mortality, and TSS, salinity, and temperature effects (Buzzelli et al., 2013b). In the STL, predicts oyster biomass production and filtration related to salinity, freshwater flow. Model incorporates temperature and TSS and impacts of oyster clearance on phytoplankton biomass (Buzzelli et al., 2013a). |
Name | Description |
---|---|
Ecological models | |
Seagrass, Mechanistic | Process-based model to examine the effects of temperature, salinity, and light attenuation (chlorophyll a, color, turbidity) on Vallisneria survival and biomass in CRE relating survival and mortality to salinity and freshwater flow (SFWMD, 2018a, Appendix A). |
CRE process-based model that predicts seagrass survival for T. Testidinum and H. wrightii as related to freshwater flow, nutrient loading, and light attenuation (turbidity, color, chlorophyll a) with gross primary production, respiration, mortality, and translocation modulated as functions of water temperature, depth, and light availability (Buzzelli et al., 2014b). | |
Process-based model that predicts seagrass S. filiforme biomass via mortality, respiration, and gross primary production as a function of salinity, water depth, water temperature, and light attenuation in the STL (Buzzelli et al., 2012). |
NOTE: BOD, biochemical oxygen demand; CBOD, carbonaceous biochemical oxygen demand; CRE, Caloosahatchee River Estuary; DO, dissolved oxygen; STL, St. Lucie Estuary; TSS, total suspended solids.
Watershed Loading and Estuarine Water Quality and HAB Toolkit
As the CERP is implemented, CERP project and Lake Okeechobee operations will need to be refined to maximize CERP goals, minimize HABs, and balance trade-offs between restoration objectives and human water resources needs (see Box 5-3). However, RECOVER (2020a) notes that current CERP performance measures are not designed to address water quality or HABs, and any future evaluation and assessment of water quality performance measures would require “predictive modeling tools not available at this time.” To confront this management challenge, observations, syntheses, and models are needed that address two major lines of investigation. First, it is necessary to quantify how cyanobacterial blooms are linked to flow and the degree to which Lake Okeechobee and future operations of the reservoirs/STAs are seeding downstream HAB events in the northern estuaries (Graham et al., 2020). Beyond flow, other site-specific conditions will favor or disfavor cyanobacterial or marine HABs and their toxic events, many of which are linked to climate change (e.g., nutrient species, carbon dioxide concentrations, temperature). The second line of investigation is the linkage of flow/salinity and water quality (turbidity, CDOM, nutrients, algal blooms) to light limitation for seagrass. These causal mechanisms need to be investigated comprehensively through observations, syntheses, and models—from Lake Okeechobee, the CERP reservoirs, and STAs (see Box 5-3) to the local watersheds and the northern estuaries themselves.
The scientific toolkit to support these investigations of environmental drivers of water quality in the northern estuaries and their impacts on HABs consists of
observations, synthesis, and predictive models. Watershed exports can be represented by observations or watershed loading models that predict surface flows and constituent loading from local contributing basins. The computed watershed loads are currently integrated with water releases from Lake Okeechobee and could be linked with detailed measurements and models on water releases from reservoirs, and STAs as they come online. Watershed loading models provide inputs to coupled estuarine hydrodynamic and water quality models (Figure 5-10). These estuarine water quality models produce spatially explicit predictions of mass balances of oxygen, inorganic and organic nutrients, and organic carbon. These in turn are associated with “compartments” of lower trophic level ecosystem models (e.g., freely dissolved or associated in the live or dead biomass of primary producers and consumers). Ocean observations or models provide forcing of hydrodynamics and water quality to represent exchanges with the ocean.
In this section, the status of the existing scientific toolkit (including observations, synthesis, and predictive models) to support these decisions linked to water quality in the northern estuaries and their contributing basins is discussed.
Status of water quality and HAB observations.
Florida has a long history of investing in water quality monitoring, supported by active local partnerships (Patino, 2014). As with many states, Florida’s HAB monitoring appears to be focused on HAB event response and routine monitoring to assess risk to human health, recreation, and drinking water5 (i.e., public health advisories). Many environmental drivers are routinely monitored (flow, nutrients, temperature) or could be derived from water quality model output, but they are not currently synchronized in time and space with inland HAB monitoring. Adding HAB response indicators to routine water quality stations may address this gap. A monitoring program has recently been reinvigorated to investigate the environmental drivers of phytoplankton community composition and harmful algal bloom species in the St. Lucie Estuary (A. Wachnicka, SFWMD, personal communication, 2020), as an example of improved monitoring. A similar program was also proposed but listed as unfunded in the Caloosahatchee River Estuary (SFWMD, 2019a). Remote sensing federal–state collaborations, such as the Cyanobacteria Assessment Network (CyAN; Coffer et al., 2020; Urquhart et al., 2017), offer historical data that could be used for analyses and modeling hindcasts or real-time or seasonal forecasting. These could support operation of the control structures in a manner that allows both water quality and quantity to be considered in adaptive management.
Predicting watershed and estuarine water quality.
Watershed loading and estuarine water quality models are routinely applied to manage nutrients
___________________
5 See https://fdep.maps.arcgis.com/apps/webappviewer/index.html?id=d62c3487e8de49f6b3a6559cdf059e14.
and eutrophication in estuaries. State investments have supported the development of a series of watershed and estuarine water quality models for the St. Lucie and Caloosahatchee basins (Buzzelli et al., 2014b,c; Tetra Tech, 2017). These tools were developed to support management decisions on nitrogen and phosphorus TMDLs and basin management action plans (BMAPs). As CERP projects affecting the northern estuaries are completed and come online, these modeling tools can help decision makers understand the effects of these projects and Lake Okeechobee operations on water quality. Water quality parameters (e.g., temperature, salinity, nutrients, turbidity, water color, phytoplankton and benthic algal biomass, dissolved oxygen) are linked to HABs (Burford et al., 2020) and are important predictors of habitat condition for oysters and seagrass (Buzzelli et al., 2013a, 2014c).
In the Caloosahatchee River Estuary and watershed, the quantitative understanding of water quality drivers is more comprehensive than in the St. Lucie Estuary. Buzzelli et al. (2014b,c) developed a box modeling approach to estimate the relative effects of water color, chlorophyll a (an indicator of algal growth), and turbidity on light availability to seagrass. A watershed loading and estuarine hydrodynamic and water quality model has recently been updated and calibrated to support refinements of the TMDLs for the estuary (Tetra Tech, 2017). Watershed nutrient and suspended sediment loads by sources and pathways have also been updated along with improvements in predictions of estuarine nutrient concentrations, dissolved oxygen, and chlorophyll a (Tetra Tech, 2017). Although these models may be sufficient to inform decisions on a revised nitrogen TMDL, application of this specific version to investigate site-specific controls on HABs or optimize CERP seagrass restoration may be limited, unless additional improvements are made. First the watershed loading model overpredicted turbidity and did not specifically predict dissolved organic carbon, which was used in the estuary water quality model as a proxy for CDOM. Moreover, the estuary water quality calibration revealed challenges with the accuracy in spatial predictions of chlorophyll a, turbidity, and dissolved organic carbon, all of which impact light attenuation—a key control on cyanobacteria dominance and seagrass habitat quality (Tetra Tech, 2017). Additional process studies are needed to tune model constants to refine the watershed loading and estuarine water quality model calibration. Monitoring and research are currently under way at the SFWMD to better understand the sources and gradients of turbidity and CDOM in both the Caloosahatchee and the St. Lucie watersheds and to evaluate how this could improve water quality predictions in the estuary.
In contrast, comprehensive synthesis and modeling to predict watershed loading and estuarine water quality in the St. Lucie Estuary is lagging behind that of the Caloosahatchee, although extensive monitoring data exist. Limited
synthesis and modeling constrains the ability to predict the water quality factors affecting HABs or suitable habitat for seagrass and oysters. The most recent hydrodynamic and water quality modeling effort occurred to support the nitrogen and phosphorus BMAP of the St. Lucie Estuary (Ji et al., 2007; SFWMD, 2018d; Wan et al., 2012), which specified the implementation plan to achieve the 2008 nitrogen and phosphorus TMDLs. Wan et al. (2012) noted issues with water quality model calibration (e.g., bias, low model skill) specifically with respect to nutrients, phytoplankton biomass, and dissolved oxygen. Turbidity and CDOM were not modeled, even though these parameters are known to play an important role in controlling primary production, respiration, and light limitation for seagrass. A watershed loading model for the St. Lucie Basin has been recently recalibrated, but the authors noted issues with spatially resolved predictions of inorganic and organic nitrogen and chlorophyll a. Again, CDOM and turbidity were not specifically simulated (SFWMD, 2018d).
Predicting HABs.
Although water quality modeling is in routine practice, understanding the mechanisms of HABs and predicting blooms from waterbody hydrodynamics and water quality is still an emerging and rapidly evolving area of science (Burford et al., 2020; Stauffer et al., 2019). Hindcasts and seasonal forecasting based on proxies of cyanobacterial biomass are most advanced for well-studied waterbodies (e.g., Lake Erie; Bridgeman et al., 2013; Obenour et al., 2014; Stumpf et al., 2012), but the science of prediction of toxic events at a whole-waterbody scale is in its infancy (Burford et al., 2020). Incremental steps are useful; empirical models can be used to refine regional or waterbody-specific risk relationships based on the probability of increasing toxic bloom events with increased chlorophyll a, nitrogen, and phosphorus (Yuan and Pollard, 2015; Yuan et al., 2014) or to support short-term forecasts of cyanobacterial blooms (Wynne et al., 2013). Building a predictive HAB modeling toolkit for the northern estuaries requires implementation of a sustained long-term monitoring and research program in both northern estuaries and their contributing basins, including CERP projects. This predictive toolkit would need to comprehensively link environmental drivers (e.g. nutrients, meteorological and hydrologic conditions) to eutrophication and HAB responses, such as remotely sensed phycocyanin (a pigment in cyanobacteria) and chlorophyll a, in situ phytoplankton community composition, cell counts, and toxin concentrations. These investments would help to identify environmental drivers of toxic HAB events and effective approaches for their mitigation.
Incremental progress has been made predicting HABs in Lake Okeechobee. The Lake Okeechobee Water Quality Model has been developed to predict eutrophication and cyanobacterial blooms. James (2016) described results modeled over the period 1983-2012 as excellent for inorganic suspended solids,
light extinction, total phosphorus, and dissolved inorganic phosphorus, but the model did not meet goodness-of-fit criteria for total nitrogen, dissolved inorganic nitrogen, chlorophyll a, and relative abundance of cyanobacteria versus other phytoplankton functional groups. James (2016) noted that process studies are needed to represent more complex representation of the full nitrogen cycle and algal (including HAB) community responses to nutrients, their variable carbon-to-nitrogen ratios, and other environmental factors.
Water quality and HAB modeling is also needed for CERP projects. This is particularly true for the C-43 and C-44 reservoirs, which are expected to hold water for extended release during the dry season, when temperatures are highest and thermal stratification could expect to set up ideal conditions for cyanobacterial blooms. C-44 is being planned to incorporate an STA, but their ability to reduce nitrogen concentrations or remove cyanobacterial cells or associated toxins is not well quantified (see also Chapter 3). Because management of cyanobacterial blooms requires management of both nitrogen and phosphorus (Gobler et al., 2016; Kramer et al., 2018), research on nitrogen cycling and its role in cyanobacterial blooms is needed to complement the long history of phosphorus research in the northern Everglades.
Climate change is already exacerbating conditions that support HABs (e.g., higher temperature, thermal stratification, drought and associated low flows and long residence time, high irradiance, high carbon dioxide concentrations; Burford et al., 2020). Given these pressures on water management, it will be essential to understand the system interconnectivity and feedbacks to be able to specify the precise environmental flow requirements of the northern estuaries, understand the trade-offs between flow, water quality, and HABs, and determine how water quality management (source reduction, treatment versus ecosystem restoration) can improve ecosystem resilience. Florida’s capacity to respond to these climate change pressures will be aided by a water quality and HAB decision support toolkit, with investments to make models increasingly mechanistic (Burford et al., 2020).
Ultimately, understanding the expected and actual effects of CERP investments on water quality and the changing trends outside of CERP management that affect estuary water quality will be essential to inform ongoing decisions about operations and the need for investments to meet CERP and non-CERP estuary restoration goals. The scientific priority is high because of the risk of CERP not meeting its goal, not to mention the risk of HAB effects on human and ecosystem health and the Florida economy. It is beyond the scope of this report to address the full list of science questions needed to inform HAB management; Florida’s Blue-Green Algae Task Force is beginning to address these issues.6 Nevertheless,
___________________
6 See https://protectingfloridatogether.gov/state-action/blue-green-algae-task-force.
silos between CERP and non-CERP efforts will only hinder progress on this issue. CERP planners need to understand the evolving science on HABs and adapt planning and operations to this understanding.
Biological Effects Monitoring, Synthesis, and Modeling
As CERP planning proceeds, decision makers will need to understand how Lake Okeechobee and CERP reservoirs/STAs can be operated to achieve seagrass and oyster goals, while minimizing HABs. As noted in the previous section, trade-offs are likely and need to be spatially quantified. Flow and salinity are also linked to management of stressors associated with climate change (e.g., sea-level rise, increased flood and drought, increased ocean acidification, temperature, HABs). To quantify these trade-offs and manage these estuaries toward improved ecosystem resilience, the CERP needs biological modeling tools that can capture the quantitative, nonlinear relationships among freshwater flows, interconnected estuarine environmental drivers, and these major biological outcomes.
Recently updated RECOVER salinity and hydrologic performance measures have been developed using estuarine numerical hydrodynamic models to establish spatially explicit flows associated with salinity ranges that are considered “optimal” for Eastern oyster (Crassostrea virginica), brackish-water, and marine seagrass (RECOVER, 2020a; Figure 5-11). The “optimal” salinity ranges were established based on experimental and field-based studies and long-term monitoring data of organismal responses to changes in salinity. This
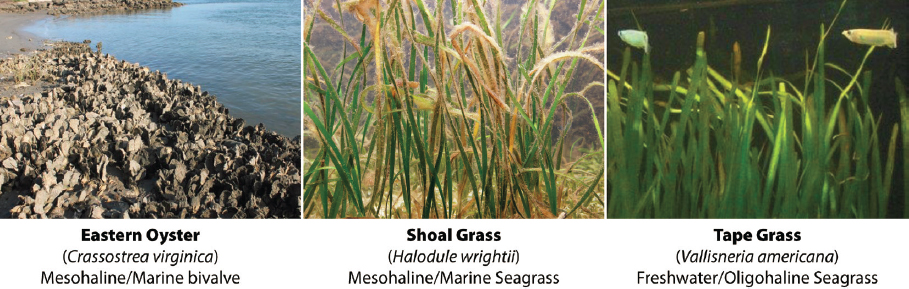
SOURCES: FWS (2017), Hans Hillewaert/Wikimedia Commons, and https://www.nps.gov/foma/learn/nature/images/Oyster-Reef.JPG?maxwidth=650&autorotate=false.
“conceptual habitat area approach” was used to query model simulations over a 50-year period in order to produce the flow envelopes that represent the maximum potential habitat for each indicator species (RECOVER, 2020a). RECOVER used these revised performance measures combined with recently updated and quantitatively validated habitat suitability index (HSI) models for its analysis of Interim Goals (due in late 2020); the Interim Goals and these updated biological models were not available for review by the committee. Previously published examples of HSI models for seagrass and oysters are empirical representations of the relationships between environmental drivers (e.g., salinity) and organism responses (seagrass extent).
These empirical models are useful as restoration planning tools, but RECOVER (2020a) acknowledges that this simplified modeling approach, which represents the “best available science,” is insufficient to optimize flow regimes for several reasons. First and most importantly, the RECOVER (2020a) conceptual habitat approach relies primarily on salinity to define potential habitat, when other factors (e.g., light availability, CDOM, nutrients, chlorophyll a, turbidity, food resources, temperature) can exert strong controls. Empirically derived HSIs, although useful for many applications, do not capture the nonlinear feedbacks that occur when organisms are exposed to multiple stressors or resources that vary over time and space (Livingston et al., 2000). Second, both the conceptual habitat approach and any empirically derived HSI average over ecologically important time steps. For example, flow–salinity relationships were analyzed based on 2-week means (RECOVER, 2020a), but extreme precipitation events can have deleterious effects on oysters and seagrass on short-term timescales of days to weeks. Third, mechanistic physiologically based models are likely to provide better predictions than empirical models for atypical combinations of drivers (e.g., sea-level rise, acidification, warming). Thus, the readiness of this biological toolkit to optimize flows for seagrass and oysters and inform management decisions that foster ecosystem resilience in light of climate change is moderate for reasons explained in detail below. The scientific priority is high, because this is a key limitation for the CERP to reach its goals and to provide clear justification for how HABs and water quality issues can be minimized, while optimizing CERP seagrass and oyster restoration goals.
Ideally, estuarine biological tools to optimize seagrass and oyster habitat would be species-specific, spatially explicit mechanistic models that depict physiological responses to highly variable temporal and spatial gradients in flow and water quality parameters (e.g., salinity, temperature, turbidity, substrate, nutrients, CDOM, phytoplankton biomass). They would be driven by output from or dynamically coupled to estuarine hydrodynamic and water quality models to predict spatially explicit biological outcomes (e.g., seagrass biomass). Because
they are mechanistic and physiologically based, these biological models can ultimately be used to evaluate trade-offs and refine restoration and adaptive management strategies as these estuaries evolve with climate change. Process-based physiological seagrass unit-modeling approaches are well established that predict above- and belowground biomass as a function of physiological stressors (salinity, temperature, root oxygen, light limitation) and resources (nutrients, carbon) (Madden, 2013; Madden and McDonald, 2010). Approaches for process-based, mechanistic oyster models are also well established (Dekshenieks et al., 2000; Fulford et al., 2007; Powell et al., 2003; Wang et al., 2008), in which oyster filtration and growth are linked to environmental conditions and food resources, including incorporation of feedback relationships (e.g., effects of oyster biodeposits on nutrient cycling; Cerco and Noel, 2007).
Important assets exist in South Florida with which to construct these more advanced biological modeling tools. The SFWMD invested in two decades of oyster and seagrass monitoring and research within the northern estuaries. Comprehensive conceptual models have been published for the Caloosahatchee River Estuary (Barnes, 2005) and the St. Lucie Estuary (Sime, 2005) and relationships among drivers are generally understood. The SFWMD has also been working to incrementally advance syntheses and empirical and process-based models with relationships to flow and salinity, based on these monitoring data for the northern estuaries.
The Minimum Flow and Minimum Water Level 2018 update for the Caloosahatchee River Estuary is the most recent and comprehensive example of a synthesis and model development across multiple biological endpoints. Mechanistic or statistical models for 11 different estuarine biological response endpoints were developed to identify minimum flow targets. SFWMD (2018a) noted that the existing monitoring data posed significant challenges to developing empirical relationships with flow and salinity, because of changes in seagrass and oyster monitoring protocols in the mid-2000s. As of 2018, RECOVER and the SFWMD reinstated an improved, intensive seagrass monitoring program in both of the northern estuaries, using more appropriate standard methods that are responsive to changing environmental conditions (e.g., Neckles et al., 2012), with greater spatial resolution (RECOVER, 2020a; SFWMD, 2019a). These are positive changes that will improve the value of the monitoring data collected, although prioritizing observations and research specifically to inform iterative mechanistic model development would expedite the development of tools to support CERP decision making.
Although no comprehensive synthesis of flow relationships with various biological endpoints has been completed for the St. Lucie Estuary (SFWMD, 2000), observations and research have been harnessed to develop seagrass and oyster
process-based models for both estuaries (Table 5-5; Buzzelli, 2011; Buzzelli et al., 2012, 2013a, 2014b). These models represent an incremental step toward an advanced biological toolkit. For example, the process-based oyster model for the St. Lucie Estuary (Buzzelli et al., 2013a) could give a spatially resolved estimate of potential habitat acreage and also inform the higher level of nutrient loading tolerated if oyster restoration goals were achieved. For seagrass, agency expertise exists to develop a more mechanistic, process-based approach for the St. Lucie Estuary, similar to that already in application in Florida Bay (Madden, 2013; Madden and McDonald, 2010). These modeling efforts could take advantage of improved seagrass monitoring by the SFWMD now under way as well as recent advances in water quality modeling to develop more spatially explicit predictions of seagrass biomass and distribution. Although freshwater flow will exert a major control on seagrass distribution, localized influences such as shoreline development, multiple uses (e.g., marinas, boating), and pollutant inputs may apply important controls on what oyster and seagrass habitat can be recovered locally.
This mechanistic, spatially explicit biological toolkit can provide important decision support to formulate a management strategy to respond to climate change. Rising sea levels will increase salinity levels in the northern estuaries and compress estuarine habitat. Acidifying and warming habitat will further stress all estuarine biota, including seagrass and oysters. Consideration should be given to incorporation of climate change stressors into the current monitoring and modeling program in order to build capacity for predictive modeling that can be used to optimize ecosystem resilience.
Summary: Integrated Decision Support Tools for the Northern Estuaries
Meeting the challenge of restoring the northern estuaries will require an advanced set of decision support tools, in combination with effective coordination and communication among scientists, water quality managers, and natural resource managers (local, state, and federal), to collectively develop, implement, and integrate these tools. These tools, namely monitoring and research, syntheses, and modeling, could be used to support quantitative understanding of trade-offs, operationalize decisions, and support long-term regional planning and coordinated management actions among CERP and other water quality and natural resources managers to enable rapid response to changing conditions under future climate change. The criteria for and status of this northern estuary decision support toolkit are summarized in Table 5-6.
TABLE 5-6 Criteria and Status of Monitoring, Research, Syntheses and Predictive Modeling Tools for the Northern Estuaries
Category | Criteria for Status of Scientific Components | Status | |
---|---|---|---|
Caloosahatchee | St. Lucie | ||
Integrated Hydrologic System | Terrestrial hydrologic basin predictions: Can capture links in the hydrologic cycle from northern everglades, WCAs, STAs, and water resource operations (including impacts of climate change) | Advanced | Advanced |
Coupled freshwater–groundwater predications to estuaries and estuary hydrodynamic models: Freshwater flows from surface and groundwater, ocean tidal forcing and WSE (water surface elevation), estuarine hydrodynamic water, salinity (including impacts on climate change) | Advanced | Advanced | |
Watershed and Estuarine Water Quality | Can predict watershed water quality including the temporal variability in water quality in releases of water from Lake Okeechobee, CERP projects as they come online (C-43, C-44 reservoirs, STAs), and local land uses as a function of external and internal drivers | Intermediate | Early Stage |
Can predict estuarine water quality, including the ability to estimate/model patterns in concentrations and mass balances of nutrients and organic carbon, production of algae and partitioning among major taxonomic groups, and the factors limiting light to seagrass. Models should be spatially and temporally resolved to investigate management options at a local scale. | Intermediate | Early Stage | |
Can predict watershed and estuarine toxic HABs as a function of environmental drivers. Simple statistical or empirical models can quantify risk relationships based on observations, while building toward mechanistic models and operational models that could be used to remediate drivers or develop rapid response management actions in response to bloom events | Emerging | Emerging | |
Biological Outcomes | Can predict seagrass biomass: Spatially explicit, mechanistic biomass models would be used to predict restored habitat acreage as a function of flow regime and target and remediate local stressors within estuarine sub-basins. Modeled biomass would respond to changes in salinity and light regimes (linked to substrate, depth, and water quality, i.e., color, turbidity, and algal abundance/nutrients) | Emerging | Emerging |
Can predict spatially explicit estimates of oyster density: Based on estuarine volume, phytoplankton turnover (as a food resource), and oyster filtration rates. Water quality models (see above) could be used to provide inputs representing phytoplankton/detrital food resources for these process-based oyster models. | Intermediate | Intermediate |
NOTES: Status ranges from emerging (observation or science components not yet implemented to capture relationships between drivers and environmental problems), early stage (basic relationships observed and reported but data gaps inhibit formulation of advanced predictive tools have not yet been addressed), intermediate (observations and research synthesized and preliminary predictive tools developed, more developments/refinements are needed), and advanced (predictive tools validated and in routine use).
SOUTHERN ESTUARIES
The southern estuaries are part of a contiguous network of coastal wetlands and estuaries that extend from Biscayne Bay through Florida Bay to the Ten Thousand Islands on the southwest coast. The high habitat diversity of the region provides many benefits including support for a broad array of aquatic life, making the region one of the most ecologically and economically important in Florida (Graham et al., 2020; Sklar et al., 2005). Biscayne Bay and Florida Bay are the largest water bodies of the southern coastal system, and the CERP envisioned a series of restoration projects to improve the quantity and distribution of their inflows. In this section, the committee describes the hydrologic and water quality changes to these ecosystems, their ecological impacts, and restoration goals for Florida Bay and Biscayne Bay. In light of continued project planning and adaptive management in this region, key decisions for water management are discussed along with the adequacy of science to support these decisions.
Biscayne Bay
Biscayne Bay (Figure 5-12) is a highly productive and biodiverse tropical marine ecosystem consisting of a series of connected, shallow lagoons, many of which open to the Atlantic Ocean. Historically, large oyster beds occurred in the nearshore zone, and water was clear with seagrass reported at water depths of 10-12 feet. Until recently, Biscayne Bay supported vast benthic communities dominated by meadows of seagrasses (e.g., Thalassia testudinum and Halodule wrightii), which contributed to its value as nursery habitat and ultimately the function and dynamics of the larger Florida Keys coral reef ecosystem (Ault et al., 2001). Biscayne Bay supports a large coral reef environment and a diversity of species, including an estimated 150 species of shrimp, crabs, sponges, and lobsters and more than 500 tropical and temperate fish species. Manatees, dolphins, alligator, and crocodiles are present, and the coastal zone and intertidal areas are a major stopover for migrating shorebirds. In an effort to protect the Bay and its diversity, an area of approximately 270 mi2 in Central and South Biscayne Bay was designated as Biscayne Bay National Park in 1982—the largest marine park in the U.S. national park system. The park protects one of the most extensive coral reef tracts in the world (Briceño and Boyer, 2010).
As a result of this diversity of habitats and species, Biscayne Bay provides a suite of ecosystem services including support of fisheries for food and recreation, protection from storms and flooding, water supply, climate regulation through carbon uptake and storage, and aesthetics (Armistead et al., 2019). These services are the basis for widespread economic activity that the citizens of Florida
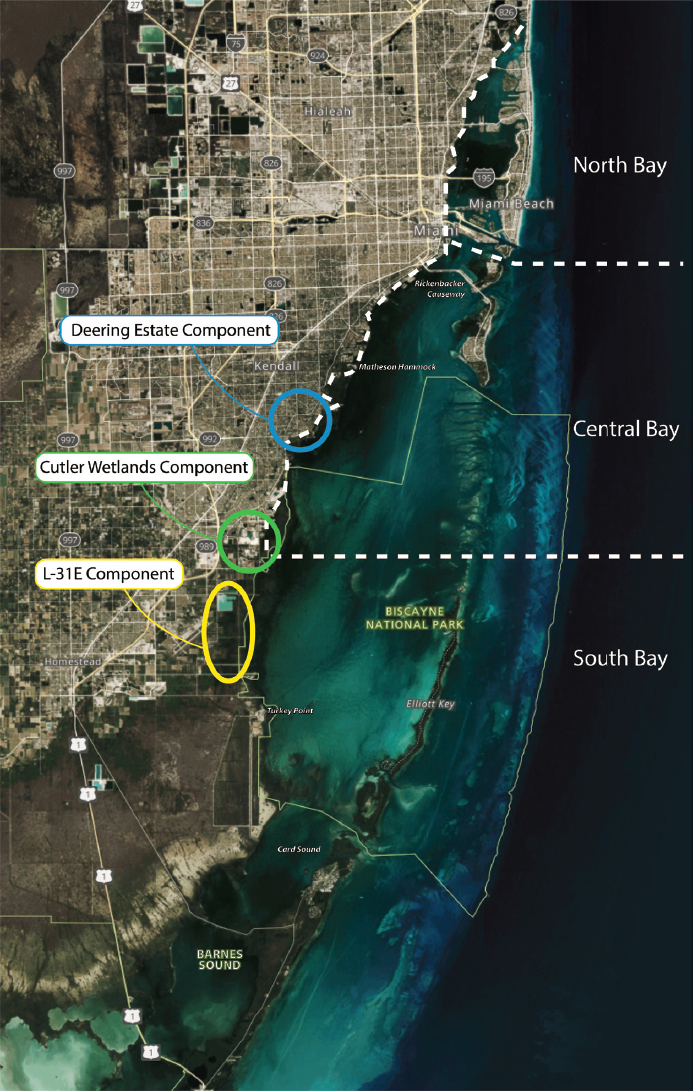
SOURCE: Google Maps.
depend on, with the value of Biscayne Bay ecosystem services estimated to be between $1.5 and $2.2 billion annually (Armistead et al., 2019).
A gradient of conditions exists from north to south along the length of the Bay. To reflect these differences, Biscayne Bay is divided into three sections: the North Bay, which is bordered by the Miami urban area (from Dumfoundling Bay south to the Rickenbacker Causeway); South Biscayne Bay, with its watershed dominated by agricultural land use (from Black Point south to Barnes Sound); and the Central Bay, a transition zone between the North and South Bays (Caccia and Boyer, 2005; SFWMD, 1995). Recent declines in water quality in North and Central Biscayne Bay have caused massive seagrass die-offs (Millette et al., 2019; RECOVER, 2019), threatening the ecological condition of the Bay and complicating the opportunities for restoration under the CERP. The following sections detail the changing characteristics of Biscayne Bay, CERP objectives, and the status of existing science and modeling tools to guide restoration decision making.
Environmental Changes and Their Ecological Effects
Structural and hydrologic changes.
Historically, Biscayne Bay was a tidal estuary that received surface-water flows over and through the Atlantic Coastal Ridge from the Everglades (Lodge, 2016). Sheet flows across coastal marshes and through numerous tidal creeks created an estuarine salinity regime of ~5-18 PSU in the fringing mangroves, tidal creeks, and the open water of the Bay (Kohout and Kolipinski, 1967; Lodge, 2016). Groundwater inflow from the Biscayne Aquifer was vital in support of the biological diversity of the Bay (Kohout and Kolipinski, 1967). Much of the groundwater inflow entered the Bay from below, causing freshwater and saltwater to mix from the bottom up, limiting the water column stratification that is common in many estuaries (Lodge, 2016). Construction of the Central and South Florida Project and other drainage projects for flood control reduced groundwater levels and shifted the groundwater divide westward from the coastal ridge into Everglades National Park (Fennema et al., 1994). Surface- and groundwater inflows were radically altered so that canal discharges and marine inflows now dominate (Wang et al., 2003); estimates are that groundwater inflows and overland flows currently represent just 10 and 6 percent of the total freshwater inflows, respectively (Briceño et al., 2011).7 The decreases in groundwater flows have resulted in a transition of Biscayne Bay from an estuary to a marine lagoon (Lodge, 2016; Wingard et al., 2004), with high and variable salinity and periodic mesohaline conditions (5-18 PSU) in areas
___________________
7 This water budget is based on inflows south of the Rickenbacker Causeway. The remainder of the freshwater budget is canal flow (44 percent) and rainfall (40 percent).
directly adjacent to the western shoreline that receive some freshwater inputs (Briceño et al., 2011). Salinities in the coastal wetlands have increased from 0 to 10 parts per thousand (ppt) under predrainage conditions to around 8-18 ppt at present (Gaiser and Ross, 2004). Urban development has also reduced and fragmented coastal habitats as the population of Miami-Dade County has grown from 12,000 people in 1910 to 2.5 million people in 2010.8
Biscayne Bay is now a fully marine system, and the impacts of climate change and accelerating rates of sea-level rise are a major concern. Over the 31 years from 1985 to 2016, sea levels have risen by 6 inches at Miami,9 while under USACE high sea-level rise projections, it may take only 15 years to rise by another 6 inches.10 The impacts of sea-level rise in Biscayne Bay are many, including increased flooding and saltwater intrusion into freshwater canals and the Biscayne Aquifer. In response, Miami has expanded its stormwater pumping system that moves water from the city into the North Bay, carrying with it debris, sediment, and other pollutants.
Water quality.
Nutrient enrichment in Biscayne Bay compounds the effects of altered hydrology. Historically, low phosphorus concentrations in flows from the Everglades resulted in a severe phosphorus limitation in the Bay’s waters (Brand et al., 1991), but concentrations of phosphorus and chlorophyll a—an indicator of algal abundance—are increasing throughout the Bay (Caccia and Boyer, 2005; Millette et al., 2019; SFWMD, 1995). Over a 20-year period, from 1995 to 2014, chlorophyll a concentrations increased significantly in all three regions of Biscayne Bay, with the largest increase occurring in the North Bay (Figure 5-13; Millette et al., 2019). Currently, increases in both phosphorus and chlorophyll a are highest in the North Bay, which is highly urbanized with restricted circulation and high population density, and in nearshore stations in the Central Bay that are close to specific canal outflows (Brand et al., 1991; Briceño et al., 2011; DERM, 2019; Gimenez, 2019; Millette et al., 2019). Sources of phosphorus include untreated urban stormwater runoff, septic tank leachate, and runoff from agricultural land (DERM, 2019). Higher groundwater levels resulting from sea-level rise are a particular threat to the function of septic tanks; by 2018, an estimated 56 percent of septic tanks in Miami-Dade County (or more than 58,000 properties with septic tanks) were compromised during storms or wet years (Miami-Dade Circuit Court, 2018), leading to nutrient inputs and high fecal coliform levels in the North Bay.11 FDEP recently verified Biscayne Bay as impaired by nutrients and high chlorophyll a levels, and a TMDL is planned.
___________________
8 See http://www.census.gov/popest/data/index.html.
9 NOAA, Station Data Virginia Key; see tidesandcurrents.noaa.gov.
10 See www.corpsclimate.us.
11 See https://floridadep.gov/dear/water-quality-restoration/content/impaired-waters-tmdls-andbasin-management-action-plans.
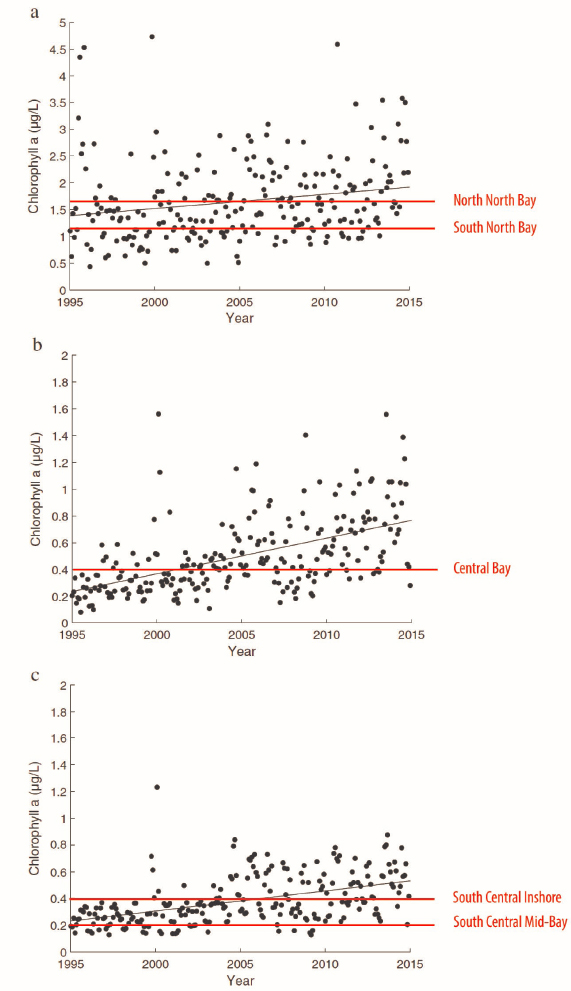
SOURCE: Millette et al., 2019.
In support of this effort, specific nutrient criteria for the different sections of the Bay have been developed (Figure 5-13; Florida Administrative Code Rule 62-302.532).
Ecological implications.
The ecological conditions of Biscayne Bay are increasingly affected by human activities that may limit the ability of the CERP to reach its goals. For example, seagrass communities in the North and Central Bay were relatively stable until 2005 when hypersalinity and increasing chlorophyll a concentration, associated with a series of algal blooms, led to extensive seagrass losses. Cumulative losses amount to 65 percent, or 21 mi2 of seagrass over the past decade, including nearly half the manatee grass (Figure 5-14; Millette et al., 2019). Specific seagrass habitat losses between 2005 and 2018 include a 77 percent decrease in the Julia Tuttle Basin, decreases of 90 percent in the 79th Street Basin, 66 to 89 percent north of Rickenbacker Causeway, and 93 percent in Barnes Sound and Manatee Bay basins (DERM, 2019; Figures 5-14 and 5-15). The die-off of seagrasses in Barnes Sound and Manatee Bay in the South Bay occurred in 2005 following Hurricane Katrina, which generated high volumes of nutrient-rich runoff and led to a multiyear algal bloom. These changes, combined with mangrove clearing during the expansion of US Highway 1 (Figure 5-12), have led to the loss of nearly all seagrasses in this area of the Bay (Rudnick et al., 2012). Few of these losses have shown any sign of recovery, and chlorophyll a concentrations remain elevated (DERM, 2019).
Currently, the nearshore seagrass community (from Matheson Hammock to Turkey Point) is dominated by shoal grass (Halodule wrightii) and turtle grass (Thalassia testudinum), with very little cover of manatee grass (Syringodium filiforme) (RECOVER, 2019). Turtle grass, a marine seagrass species that is intolerant of brackish conditions, is now dominant in many areas where seagrasses are present (DOI, 2006). The co-occurrence of Halodule and Thalassia is described as a desired goal of the CERP and was found, on average, at 58 percent of monitoring sites from 2008 to 2017. Estimates of cover (i.e., the area occupied by a species per unit area) for all these species is low, ranging from 17.1 percent for Halodule, 9.1 percent for Thalassia, and only 0.2 percent for Syringodium over the period of record (RECOVER, 2019), illustrating the tenuous hold that seagrasses have in Biscayne Bay.
For Biscayne Bay overall, Millette et al. (2019) predict a regime shift from a system with clear water and extensive seagrass meadows to a murky system dominated by phytoplankton with reduced benthic nursery habitat. The conditions leading to this tipping point are worsening; phosphorus and chlorophyll a levels are increasing and the system response is exacerbated by other stressors related to climate change such as salinity and increasing water temperatures (Miami-Dade Circuit Court, 2018; Millette et al., 2019). The possibility of sea-
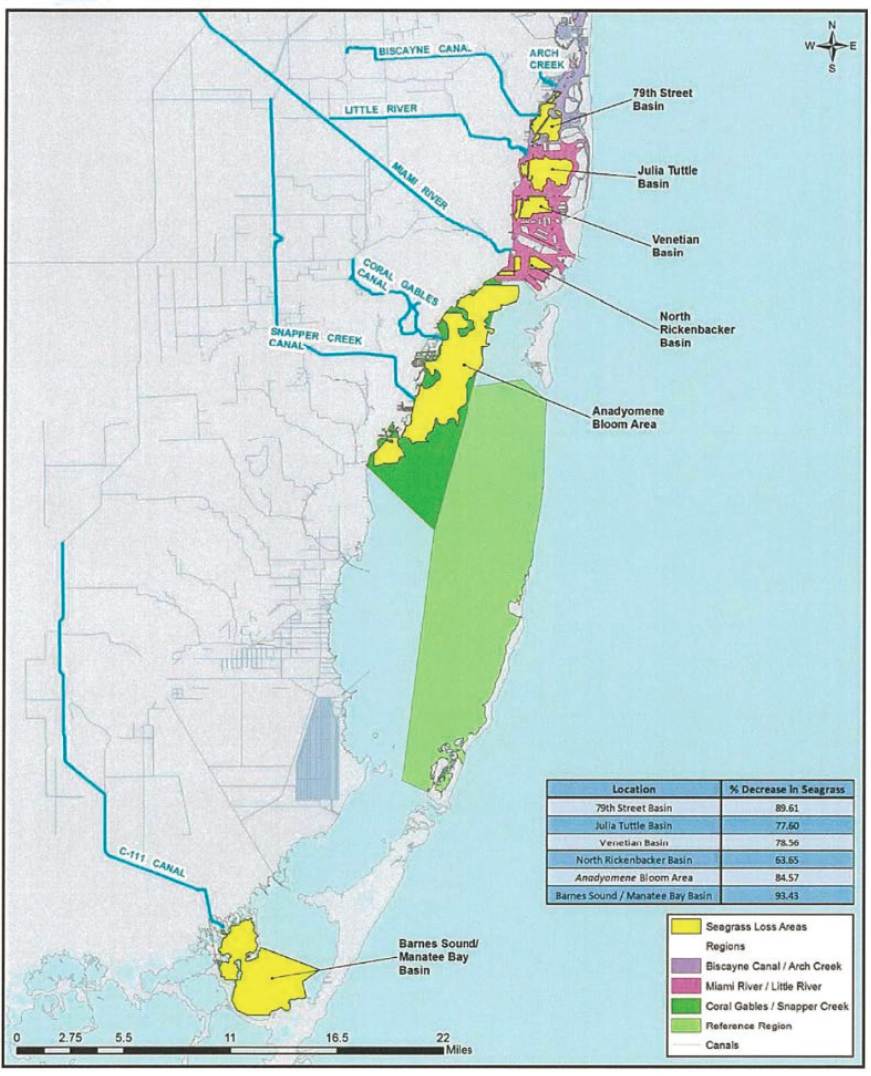
SOURCE: Gimenez, 2019.
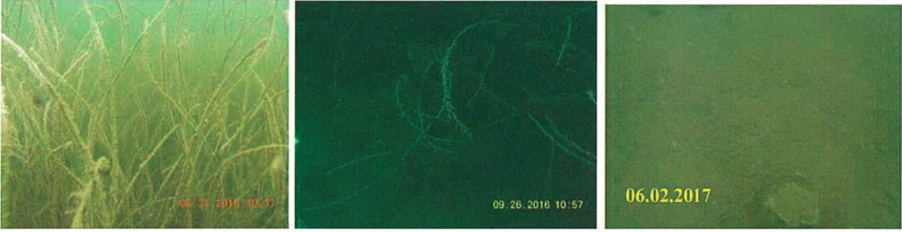
SOURCE: DERM, 2019.
grass recovery is limited; RECOVER (2019) considers the future of Biscayne Bay’s seagrass meadows as “bleak.”
Increasing salinity associated with sea-level rise and a lack of freshwater inflows affects not only seagrass but also animals who depend on mesohaline conditions, including the oyster (Crassostria virginica), American crocodile (Crocodylus acutus), spotted seatrout (Cynoscion nebulosus), silver perch (Bairdiella chrysoura), and mojarras (Eucinostomus spp.) (Bellmund et al., 2004). For these and other species, abundance decreases rapidly as salinity increases (Figure 5-16; Serafy et al., 1997). Estuarine fish species, such as red drum and black drum, have been replaced with euryhaline and marine species (McManus et al., 2014; Serafy et al., 2003). In the nearshore zone, the persistence of mesohaline conditions remains far from CERP targets, with the maximum duration of consistent mesohaline conditions ranging from 3 to 20 days in the wet season (target is 34 days) and 5 to 36 days for the dry season (target is 78 days) (RECOVER, 2019). Biscayne Bay National Park developed its own salinity targets for the western zone of the Park that are more restrictive than the CERP targets, with an overall standard that salinities should never exceed 30 PSU, and specific targets of 15-25 PSU for all of March through August, and less than 20 PSU in September through October (the late wet season). Biscayne Bay National Park also set a target for the coastal mangrove zone in the park of 0-5 PSU. Without more freshwater inflow to Biscayne Bay, the conditions will remain insufficient to support species adapted to mesohaline conditions.
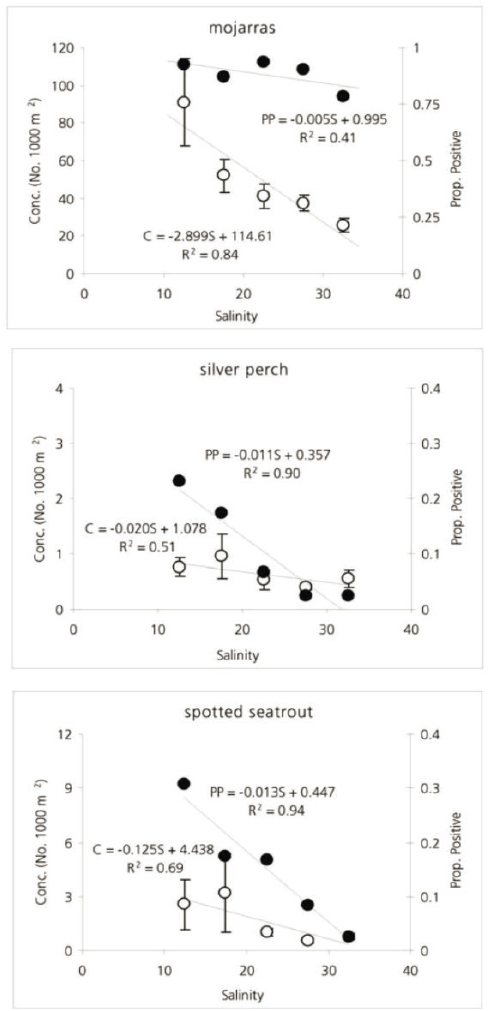
SOURCE: DOI, 2006.
Restoration Goals and Expected Effects of Planned CERP Projects
The 2007 Interim Goals Agreement (USACE et al., 2007) describe the early qualitative hydrologic and ecological expectations from the CERP for Biscayne Bay:
- Increase freshwater flows;
- Reduce the intensity, duration, frequency, and spatial extent of high-salinity events, reestablish low-salinity conditions in mainland nearshore areas, and reduce the frequency of and rapidity of salinity fluctuations resulting from pulse releases of freshwater from canals; and
- Increase densities of juvenile shrimp.
In addition, RECOVER monitors a set of indicators including seagrass (species occurrence, cover, density), chlorophyll a concentration (as a measure of phytoplankton blooms), characteristics of the mangrove fish community (species frequency of occurrence, density), epifauna adapted to mesohaline conditions including juvenile pink shrimp density (e.g., Browder and Robblee, 2009), and crocodile growth rates (RECOVER, 2019).
Yellow Book projections for Biscayne Bay.
To achieve these goals, the CERP was envisioned in the Yellow Book to improve the distribution of existing flows and provide additional water supply (77,000 AF per year—a 6 percent increase in total freshwater inflows [including canal discharges] compared to existing conditions12). These hydrologic improvements were expected to “create conditions that will be conducive to the reestablishment of oysters and other components of the oyster reef community” and “reestablish productive nursery habitat all along the shoreline” in Biscayne Bay (USACE and SFWMD, 1999). The CERP was also expected to rehydrate coastal wetlands and reduce point-source freshwater discharges to Biscayne Bay.
The lack of baseline information on predrainage conditions has limited the development of CERP freshwater flow targets for Biscayne Bay (McManus et al., 2014; RECOVER, 2011a). The Yellow Book acknowledged that a detailed analysis was needed to define specific hydrologic targets considering the feasibility of potential water sources, although this feasibility study was never completed. DOI (2008) estimates that at least 440,000 AF per year in additional freshwater flows are needed to meet salinity (<30 ppt) and ecological targets in the nearshore area associated with the National Park (between the S-22 and S-197 structures). DOI (2008) states that the bulk of flows are needed during the late dry season to early wet season—a time when water availability is typically extremely limited.
___________________
12 Relative to 2004 water budget (SFWMM v5.4); see NRC, 2005.
To date, freshwater flow targets for Biscayne Bay from the CERP and a plan to meet those targets have not been established.
Expected effects of planned CERP projects.
Two current CERP projects offer benefits for Biscayne Bay: the Biscayne Bay Coastal Wetlands (BBCW) Phase 1 Project, now under construction (see Chapter 3), and the Biscayne Bay and Southeastern Everglades Ecosystem Restoration (BBSEER) Project, which launched its 3-year planning process in 2020 (USACE and SFWMD, 2020b). The BBCW Project was divided into two phases to make incremental progress without near-term sources of new water. Phase 1 (see Chapter 3) diverts existing canal flows through the coastal wetlands to improve the salinity distribution in the coastal wetlands. The project is expected to rehydrate about 400 acres of freshwater wetlands and improve mesohaline conditions in at least 6,300 acres of saltwater wetland. The project would also reduce salinities in at least 2,900 acres of nearshore habitat by diverting an average of 59 percent of the annual coastal structure discharge into coastal wetlands (USACE and SFWMD, 2012). The planned diversions of canal water through the existing coastal wetlands are also anticipated to provide water quality benefits such as a reduction of 50 percent of the projected future nitrate load to Biscayne Bay (162 metric tons per year), although water quality improvement was not an explicit design objective of the project (USACE and SFWMD, 2012). Although there have been some improvements in salinity from BBCW Phase 1 to date, benefits have been limited by a lack of available freshwater (see Chapter 3). Overall, Phase 1 benefits are focused toward improved conditions in the coastal wetlands using existing flows, with relatively modest benefits to nearshore habitats, given the lack of additional water supply.
The BBSEER Project is envisioned as a regional planning effort that encompasses a number of projects from the Yellow Book, including additional elements from the BBCW Project not covered in Phase 1 (including in the Model Lands in South Biscayne Bay) and the eastern components of the C-111 Spreader Canal Project. It may also consider wastewater reuse in Miami-Dade County and Lake Belt storage features proposed in the Yellow Book (USACE and SFWMD, 1999). The specific benefits of BBSEER, which will be determined in the project planning process, will likely depend on the availability of new freshwater inflows.
Implications of Environmental Issues for CERP and Non-CERP Efforts
During the ongoing implementation of BBCW Phase 1, eutrophication of this historically oligotrophic system has increased, particularly in the North Bay (Millette et al., 2019; Figure 5-13). As with the northern estuaries, the CERP did not consider the potential impacts of nutrient enrichment and algal blooms when
setting restoration goals for Biscayne Bay. Without substantial new non-CERP efforts to address water quality, the complications of eutrophication may affect progress toward CERP ecological goals, not only for chlorophyll a targets but also in reestablishing nearshore nursery habitat for fish and other fauna (RECOVER, 2019). Increasing nutrient concentrations and algal blooms are hypothesized to have pushed the system toward a tipping point for a full-scale regime shift to an ecosystem dominated by algae and the loss of seagrass beds in the open waters of the Bay (Millette et al., 2019). Crossing such a threshold would be catastrophic for Biscayne Bay and could make the CERP’s ecological objectives difficult to achieve.
Key Questions for Decision Makers on Restoration of Biscayne Bay
As with the other South Florida estuaries, there are key management questions that must be answered to make sound decisions on Biscayne Bay restoration. For example, what is the timing, magnitude, and spatial distribution of freshwater inflows needed to meet restoration goals? Both federal and state programs are at work on water quality issues in Biscayne Bay, but they often work independently in the pursuit of common goals. It is vital to address the issue of water quality (a state responsibility) as a driver of Biscayne Bay conditions to meet the CERP targets for chlorophyll a and seagrass. Decisions will be needed to respond to changes in external drivers associated with climate change that will foster the long-term resilience of the system, and this will take cooperation across many groups working in Biscayne Bay. Because management decisions inevitably involve trade-offs, managers will need a sound set of integrated science tools to inform questions such as those posed in Box 5-6.
Science Support for Restoration Management Decisions in Biscayne Bay
Science-based tools for decision making are part of a valuable toolkit to ensure decisions are informed by an understanding of the trajectories of ecosystem response to management actions and environmental change. Climate change impacts are increasing, and nutrient inputs from an increasing human population are fundamentally restructuring the Bay’s drivers and ecological responses. A combined set of conceptual models, monitoring data, synthesis, and models (see Figure 5-17 and Table 5-7) can characterize and quantify the relative effect of the quantity and timing of freshwater delivery and water quality on CERP restoration targets, while separating those effects from other external and internal drivers and feedback mechanisms that also contribute to biological responses. Without this understanding, the accumulation of monitoring data
leaves efforts to restore Biscayne Bay “data rich and information poor.” In this section, the committee reviews the status of this science relative to key management questions aimed at informing CERP science priorities.
Hydrologic science and modeling tools.
Although increased freshwater flows to improve nearshore salinity in Central and South Biscayne Bay are a primary goal for the CERP, no quantitative, seasonally variable goals for freshwater flow delivery to meet restoration targets have been established (RECOVER, 2011a). One challenge for restoration of Biscayne Bay is that hydrologic and biological data on the predrainage system are almost entirely lacking, making identification of restoration flow targets challenging (McManus et al., 2014). Without this understanding, modeling tools are essential to evaluate the conditions and flows necessary to generate the desired ecological responses and to predict expected outcomes from project alternatives. This is also needed to understand the effects of shifting baseline conditions expected under a chang-
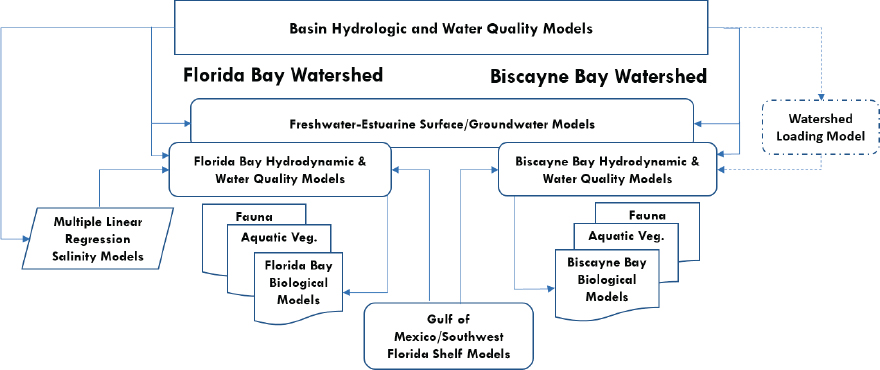
TABLE 5-7 Examples of Hydrologic, Hydrodynamic, Water Quality, and Ecological Models Applicable to Florida Bay and Biscayne Bay
Name | Domain | Description |
---|---|---|
Hydrologic models | ||
Regional Simulation Model (RSM) and Natural System RSM (NSRSM) | Everglades and Lower East Coast | The RSM simulates surface- and groundwater hydrology for current or future (RSM) or predrainage (NSRSM) conditions in response to historic climate records and data. The model outputs water levels and flows from canals, water control structures, local topography, and storage reservoirs, etc. It simulates the movement and distribution of water in conjunction with the coordinated operation of canals and structures (Bras et al., 2019; SFWMD, 2005) |
Hydrologic Engineering Center River Analysis System (HEC-RAS) | Everglades | A hydrodynamic model that simulates the channel flow regimes of canals, rivers, and channels |
Biscayne and Southern Everglades Coastal Transport (BISECT) | Florida Bay, Biscayne Bay | Evaluates drivers of South Florida surface- and groundwater water and salt budgets to evaluate seepage barrier efficacy and alternative water-management practices and sea-level rise by combining the Tides and Inflows to the Mangrove Everglades (TIME) and FTLOADDS simulator (Swain et al., 2019) |
Name | Domain | Description |
---|---|---|
Hydrodynamic and water quality models | ||
Flux Account for Tidal Hydrology at the Ocean Margin (FATHOM/BAM) | Florida Bay | Spatially explicit 54-basin box model that simulates the Florida Bay water and solutes in response to runoff, climate, tides, and the topography of the Bay by tracking mass-balance of water, salt, nitrogen and phosphorus (N&P), dissolved oxygen (DO), and heat |
Curvilinear Hydrodynamics in 3-D (CH3D) | Biscayne Bay | 3D estuarine hydrodynamic model with time-varying salinity and temperature |
Multi-Dimensional Sediment (TABS-MDS) | Biscayne Bay | 2D finite element hydrodynamic and salinity model intended to support CERP freshwater flow scenarios |
Biscayne Bay Simulation Model (BBSM) | Biscayne Bay | 3D density-dependent flow model designed to evaluate the effects of surface- and groundwater flows and redistribution on salinity |
Environmental Fluid Dynamics Code (EFDC) | Florida Bay | A 3D estuarine hydrodynamic model that transports salinity, heat, sediments, and toxic contaminants (e.g., metals or organics). The EFDC integrated water quality model HEM-3D was not completed for Florida Bay |
South Florida Hybrid Coordinate Model (HYCOM) | Florida Bay | A coastal hydrodynamic model of South Florida coastal seas developed to provide boundary conditions for the EFDC Florida Bay model |
Multiple linear regressions | Florida Bay | Multiple statistical models relating southern Everglades hydrologic (e.g. water level), marine, and weather data to predicted salinity at 37 estuarine index areas of Everglades National Park (Marshall et al., 2011) |
Biscayne Bay Box Model | Biscayne Bay | Water quality box model that estimates the long-term average N&P concentrations and loads in the Bay based on total phosphorus and dissolved in organic nitrogen loads from canals, ungauged surface-water, groundwater, atmospheric, and Atlantic Ocean contributions |
Ecological models | ||
Seagrass Ecosystem Assessment and Community Organization Model (SEACOM) | Florida Bay | SEACOM is a mechanistic, physiological unit model that predicts seagrass community type response to salinity and water quality, climate and climate change on seagrass distribution, species composition and habitat suitability for higher trophic levels; used to evaluate restoration alternatives (Madden et al., 2016) |
Seagrass Habitat Suitability Index | Biscayne Bay | Statistical seagrass habitat suitability models that predict T. testudinum and H. wrightii as a function of light, depth, salinity, and temperature (Santos and Lirman, 2012) |
Seagrass Discriminant Function model | Florida Bay | Florida Bay statistical models that predict occurrence of eight seagrass community types based on FATHOM-predicted water quality, including total organic carbon, nitrate, ammonium, total phosphorus, and salinity (Herbert et al., 2011) |
Crocodile Habitat Suitability Index | Florida Bay | Predicts habitat based on crocodile growth and survival, which is a function of salinity and prey biomass (Mazzotti et al., 2009) |
Shrimp statistical models | Biscayne Bay | Predict pink and grass shrimp as a function of salinity, water temperature, depth, and seagrass (Zink et al., 2017) |
ing climate. Climate change and sea-level rise now present new challenges for the feasibility of meeting restoration salinity targets (Graham et al., 2020), and these impacts need to be understood in order to inform restoration investments that will provide long-term benefits.
Freshwater flows to Biscayne Bay include groundwater discharge from the Biscayne aquifer, which extends westward into Everglades National Park, and surface-water inflows from canals supplied with water released from the Everglades ecosystem as well as inputs from local watersheds. Attainment of nearshore restoration goals will require modeling of the interactions of saltwater and freshwater from both surface- and groundwater flows to evaluate the potential effects of the CERP across a range of conditions. The Regional Simulation Model for the Everglades and Glades Lower East Coast Service Area (RSM-GL) can be used to simulate overland flows and water management to the coastal region of Biscayne Bay, but it is entirely a land-based model not suited for predictions of coastal zone conditions. The recent Biscayne and Southern Everglades Coastal Transport (BISECT) model (Swain et al., 2019) simulates surface-water and three-dimensional groundwater flow and exchanges between these flow regimes. The model, based on the code of the Flow and Transport in a Linked Overland/Aquifer Density Dependent System (FTLOADDS) (Wang et al., 2007), also simulates groundwater and surface-water salinity and temperature, based on hydrologic data from 1996 to 2004. BISECT supports evaluation of the effects of water management changes combined with sea-level rise scenarios on South Florida hydrology. The model domain includes coastal zones, but the primary focus is to answer questions about hydrologic conditions on land and in tidal wetlands, rather than conditions within the Bay itself. Calibration of the model used extensive hydrologic data available from Everglades National Park, including Florida Bay, but did not employ flow data from surface discharges to Biscayne Bay. As such, the performance is much better for Florida Bay than Biscayne Bay. However, this model has the potential to fill an important gap in the southern Everglades coastal region, bridging between the land-based hydrologic models (e.g., RSM-GL) and estuarine hydrodynamic models in the coastal region where the RSM performs poorly (Mills et al., 2019). It also provides a tool for more effectively capturing the effects of sea-level rise on coastal areas. BISECT model development relied on long-term monitoring data that refined the understanding of the relationships between factors such as (a) the rates of water flow in canals, marshes, and aquifers; (b) the nature of freshwater and saltwater mixing in surface waters; and (c) the rates of freshwater and saltwater exchange between surface water and groundwater in both onshore and offshore areas. However, the accuracy of the model remains limited by the lack of monitoring data in some areas, prompting calls, for example, for increased monitoring of groundwater
levels (Mills et al., 2019). Overall, models that link inland watersheds with the hydrodynamics of estuarine and coastal waters remain insufficient to address fine-scale spatially explicit water distribution issues, groundwater–surface water exchange, saltwater intrusion, and the resulting effects on biota. Improving model performance will depend on the integration of additional data (e.g., geomorphology, water levels) to capture these biophysical feedbacks. To date, BISECT has not been approved for use in CERP planning.
Models that quantitatively link surface- and groundwater inflows to nearshore salinity in Biscayne Bay are essential to CERP planning and near-term decision making. Salinity monitoring in the Bay has been foundational to estuarine hydrodynamic studies that have solidified an understanding of Biscayne Bay’s hydrodynamic circulation and links to freshwater flow. Modeling studies have quantified surface-water and groundwater inputs into Biscayne Bay (e.g., Langevin et al., 2001), and 2D and 3D hydrodynamic circulation models exist (Brown et al., 2003; Wang et al., 2003). The Biscayne Bay Simulation Model (BBSM) is a three-dimensional density-dependent flow model designed to evaluate the effects of surface- and groundwater flows and redistribution on salinity. This model was recently revised to improve its ability to predict salinity along the shoreline, in part by adding a groundwater component that had been omitted from the previous model version because of uncertainties about groundwater flows. In this most recent model update (BBSMv4), the predicted salinity values more closely matched the observed distributions of nearshore salinities, and the model was able to reproduce observed seasonal salinity patterns, with lower salinity during the wet season and higher salinity in the dry season. However, the improvements in model performance were attributed to a substantial increase in simulated groundwater flow volumes, which were required to more accurately predict nearshore salinity during portions of the year, suggesting that the existing estimates of groundwater flow to Biscayne Bay (e.g., Stalker et al., 2009) may be underestimated (Stabenau et al., 2015). Model improvements have increased the understanding of nearshore salinity patterns, but the relationship between various freshwater inflows and salinity are not fully understood.
Understanding the linkages between water quality and ecological response.
Many of the current ecological issues facing Biscayne Bay, particularly in the North and Central Bay, are a result of increasing nutrient concentrations (phosphorus and nitrogen), and elevated chlorophyll a concentrations that have led to seagrass die-offs. Currently, these issues are most severe in the open waters of the Bay (i.e., beyond the nearshore zone) that is not the focus of the CERP. However, the Bay’s deteriorating conditions have an impact on the nearshore zone and may limit the ability of the CERP to meet the restoration targets that have been established. To understand the potential implications of changes in
water quality to CERP goals for Biscayne Bay, efforts are needed that connect monitoring data, synthesis, and hypotheses with linked hydrodynamic and water quality models that, in turn, will inform ecological response models that predict recovery trajectories relative to CERP performance targets (e.g., seagrass diversity, cover, and density; chlorophyll a concentration).
A strong monitoring effort is ongoing in Biscayne Bay to support this objective. The Integrated Biscayne Bay Ecosystem Monitoring and Assessment Program13 is a strategic multiagency monitoring effort that produces quantitative data on some of the key RECOVER systemwide indicators that have quantitative performance targets (Lirman et al., 2014; RECOVER, 2019). Together with monitoring from partner agencies, these monitoring data are instrumental in documenting salinity, nutrient concentrations, and eutrophication in the Bay and the loss of large areas of seagrass.
In addition to the limitations of hydrodynamic models to predict spatially explicit salinities (discussed in the previous section), the lack of water quality models, both in the watershed and in the estuary, is a key gap in advancing restoration in Biscayne Bay. A nutrient box model (Marshall and Nuttle, 2011) was developed to estimate nutrient loads from watershed sources using mass-balance calculations, with estimates of long-term average nutrient concentrations for total phosphorus, ammonium, nitrate-nitrite, and dissolved inorganic nitrogen. However, the model has limited success in describing the variability in nutrient concentrations, with predicted values of total phosphorus 17 to 44 percent higher than measured values, and predicted nitrate-nitrite values 1.5 and 4 times higher than measured values (Marshall and Nuttle, 2011). The latter was attributed to losses due to denitrification that were not accounted for in the model. The box model was used to inform development of the Biscayne Bay nutrient criteria (Briceno et al., 2011); recently Biscayne Bay was put on the 303(d) list for impaired water quality due to elevated chlorophyll a (FDEP, 2017). The TMDL is in the planning stages, but will provide the impetus needed to develop a more comprehensive synthesis and development of water quality modeling capabilities. This water quality model could be used as input to predict possible CERP outcomes for seagrass and other biota under scenarios of freshwater flow and various water quality trajectories.
The development of ecological response models for Biscayne Bay has lagged, particularly in comparison to efforts for Florida Bay. The large seagrass die-offs in the north and central portions of the Bay demonstrate the need for models that facilitate a deeper understanding of the drivers of nutrient loading into the Bay, corresponding ecological responses, and potential interactions
___________________
13 See https://marine-biology-ecology.rsmas.miami.edu/research-themes/centers-and-labs/benthicecology-coral-restoration-lab/ibbeam/index.html.
with sea-level rise. A Biscayne Bay conceptual ecological model (Browder et al., 2005) describes the salinity regime and links between stressors and key ecosystem attributes, but subsequent development of quantitative ecological models has been limited. Currently, there is a seagrass HSI model (Santos and Lirman, 2012), with presence/absence predictions based on light, depth, salinity, and temperature. However, the relative contributions of light limitation from water color, turbidity, and chlorophyll a are not specifically distinguished in this seagrass HSI, limiting the model’s utility to integrate CERP restoration discussions with water quality management. More recently, statistical models have been developed (McManus et al., 2014; Santos et al., 2020), including work linking algal blooms to seagrass extent that demonstrated the ability of algal blooms to fragment seagrass patches, significantly altering seagrass community structure (Santos et al., 2020). Continuing work is needed to develop the toolkit for the ecological response of Biscayne Bay that will inform management decisions, such as the optimal flows needed for the recovery and persistence of seagrasses. As with the northern estuaries, it is likely that more mechanistic, physiologically based models that can predict ecological outcomes under conditions that have not yet been experienced (e.g., sea-level rise, warming) will be needed to address questions about system response to the novel set of environmental conditions that climate change will bring.
In the long term, an integrated modeling approach, coupling hydrology, water quality, hydrodynamic, and seagrass or other ecological models, is needed to link CERP freshwater inflows to the RECOVER performance indicators and to identify thresholds and tipping points in the system that may lead to irreversible changes (for an example, see Box 5-7). Future investments in this initiative would provide an important management tool for decision makers trying to identify CERP and non-CERP management strategies necessary to understand the tradeoffs and relative costs and benefits among CERP projects affecting the southern coastal systems to meet restoration goals. For example, both Biscayne Bay and Florida Bay need additional freshwater flows, but plans to send water south will have differential benefits for each ecosystem. Modeling tools that weigh differential benefits can provide information that will make clear the consequences of possible management decisions.
Florida Bay
Florida Bay is a large, shallow marine lagoon, bounded to the north by the Florida peninsula and to the south and east by the Florida Keys. The western side is a relatively open connection to the southwest Florida shelf, and exchanges occur mainly through physical forcing via winds and tides (Wang et
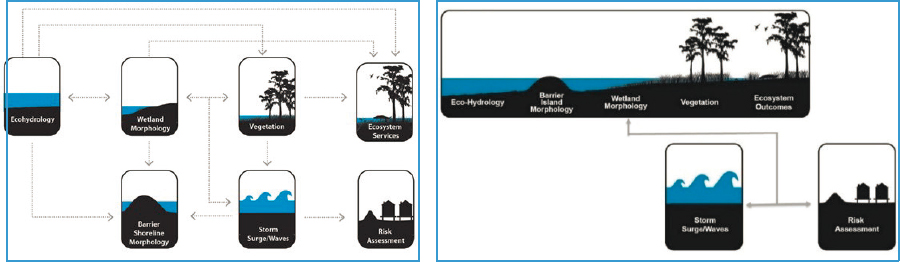
SOURCE: https://coastal.la.gov/our-plan.
al., 1994). Most of its approximately 2,200 km2 is located within the boundaries of Everglades National Park, and its unique geomorphological structure supports a mosaic of habitats, including freshwater marshes, mangroves, and abundant seagrass beds. Living organisms produce calcium carbonate (marl) sediments that form the base of the shallow bay along with extensive mud banks, which are generally exposed at high tide and divide the bay into a series of basins (Figure 5-19). Each basin has its own physical characteristics, providing a range of unique habitats that support many plants, invertebrates, fishes, birds, mammals, and reptiles, including several threatened and endangered species (e.g., the Florida manatee) and species of special concern (e.g., the roseate spoonbill).
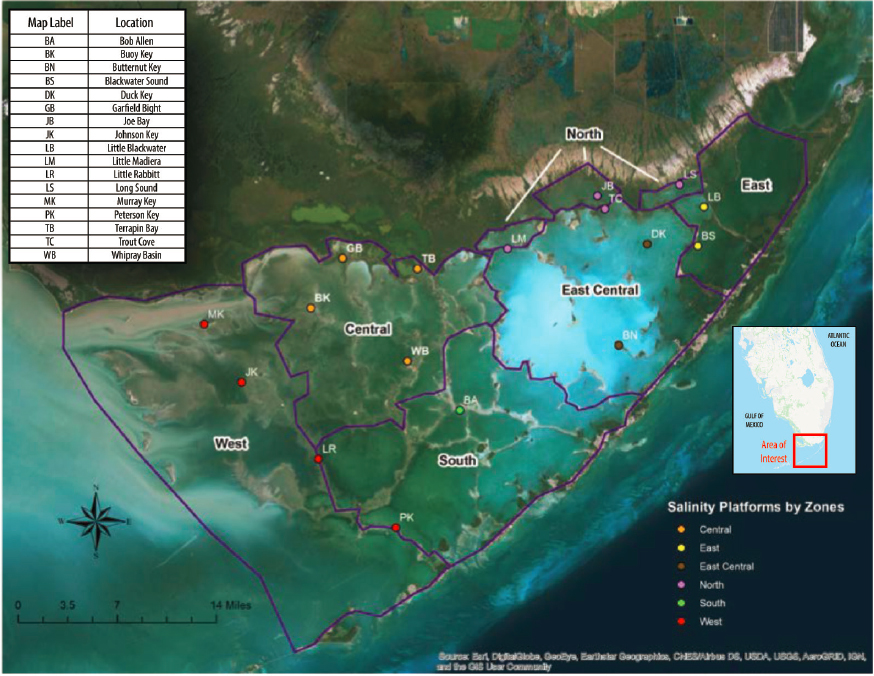
SOURCE: Modified from RECOVER, 2019.
Circulation is complex; mud banks on the western side and the Florida Keys to the southeast significantly dampen tidal influences in the interior of the bay from the Gulf of Mexico and the Atlantic Ocean. This restricted circulation also makes Florida Bay prone to extremes of salinity, temperature, and reconfiguration of sediments by storms and other factors.
Environmental Changes and Their Ecological Effects
Hydrologic changes.
As discussed in Chapter 2, drainage and flood control in the Everglades ecosystem for agricultural and urban development has vastly reduced freshwater flows to Florida Bay. Using paleoecologic data to improve existing models of predrainage conditions, Marshall et al. (2020) estimated that predrainage flows in Taylor Slough were approximately three times the recent observed flows (between 1990 and 2000) and predrainage Shark River Slough flows were approximately twice the recent observed flows. Today, the largest single source of freshwater into Florida Bay is direct rainfall over the Bay itself; for the period from 1970 to 1995, rainfall represented greater than 90 percent of all freshwater inputs to the Bay (Nuttle et al., 2000). In addition, the spatial distribution of freshwater inflows across the coastline have also changed. Current freshwater flows into the Bay are primarily from Taylor Slough (see Figure 4-2); flows from Shark River Slough that travel via currents into Central and East Central Florida Bay are minimal, typically occur only in the wet season, and have limited spatial influence (SFWMD, 2016b). Some additional flow can occur via another route; when water levels in Shark River Slough are high enough, the wet prairie area of the Rocky Glades (an area of karst that separates Shark River Slough from Taylor Slough and is seasonally flooded) may become inundated, and water flows south into Taylor Slough (Marshall et al., 2020). Under predrainage conditions, this flow would have contributed freshwater to North and Central Florida Bay (Figure 5-19).
With reduced freshwater inflows, and episodic high flows, the shallow coastal basins can experience significant excursions in salinity. Salinity in Florida Bay’s coastal basins ranges from near zero in times of heavy rainfall and large freshwater inflows to above 60 ppt during conditions of drought and high evaporation (Stabenau and Kotun, 2012). Under highly localized precipitation events, both hypersaline and hyposaline conditions can exist simultaneously in different basins across the Bay. In 2015, Florida Bay experienced a 16-month, localized rainfall deficit, during which the Taylor Slough watershed received 25-35 inches of direct rainfall—the lowest total for any part of the SFWMD’s 16-county region (SFWMD, 2016b). As a result of the rainfall deficit, salinity in the central coastal basins soared to more than 70 ppt, the highest recorded salinities in 68 years
of record (Park et al., 2016). Historically, freshwater flow from the Everglades, including Shark River flows that diluted Florida Shelf salinities in the Gulf of Mexico, maintained brackish conditions (< 30 ppt) over the entire bay and mitigated the development of hypersalinity in the northern Bay (Marshall et al., 2014) (Table 5-8). Under predrainage conditions, average salinities in North Florida Bay and northern portions of Central Florida Bay were less than one-third the salinity of seawater.
Water quality.
Plant communities in most of Florida Bay are phosphorus limited because of very high nitrogen-to-phosphorus ratios in freshwater runoff, a long residence time for water, high rates of primary production, and carbonate sediments that sequester phosphorus (Figure 5-20; Fourqurean and Zieman, 2002; Fourqurean et al., 1992, 1993). The largest source of phosphorus to Florida Bay comes from the Gulf of Mexico, which stimulates seagrass growth in western, central, and southern portions of Florida Bay. Rudnick et al. (1999) estimated the phosphorus and nitrogen inputs into Florida Bay, and determined that watershed sources were minor for the Bay as a whole; the freshwater Everglades (Taylor Slough and the C-111 watershed plus Shark River Slough) contribute approximately 3 percent of all phosphorus inputs and 12 percent of all nitrogen
TABLE 5-8 Comparison of Observed and Modeled Paleo-Based Salinities in Florida Bay
Region and Zone in Florida Bay | Map ID | Station | Observed Mean Salinity | Mean Paleo-Salinity | Salinity Difference (Obs – Paleo) |
---|---|---|---|---|---|
Northern margin (zones 1 & 5) | TB | Terrapin Bay | 23.6 | 3.5 | 20.1 |
GB | Garfield Bight | 28.9 | 10.3 | 18.6 | |
LM | Little Madeira | 23.8 | 8.2 | 15.6 | |
TC | Joe Bay | 15.4 | 2.7 | 12.6 | |
Eastern (zone 2) | BN | Butternut Key | 31.3 | 17.7 | 13.6 |
DK | Duck Key | 29.0 | 16.8 | 12.2 | |
Central & Western (zones 3, 4, 6) | BA | Bob Allen | 33.2 | 21.1 | 12.1 |
BK | Buoy Key | 32.8 | 22.2 | 10.6 | |
JK | Johnson Key | 35.3 | 27.0 | 8.3 | |
MK | Murray Key | 33.0 | 24.8 | 8.2 | |
LR | Little Rabbit | 34.4 | 27.3 | 7.1 | |
PK | Peterson Key | 35.8 | 30.5 | 5.3 |
NOTES: See Figure 5-19 for locations. Based on output from the linkage between linear regression models and paleoecological data. Time periods for observed data range from 1998 to 2002 (see Marshall et al., 2009). The simulated paleo-based salinities are based on estimates for circa 1900 AD segments of the cores used to adjust existing hydrologic models that incorporate the 1965-2000 climate data.
SOURCE: Wingard, 2017. Reprinted with permission; copyright 2017, Springer Nature.
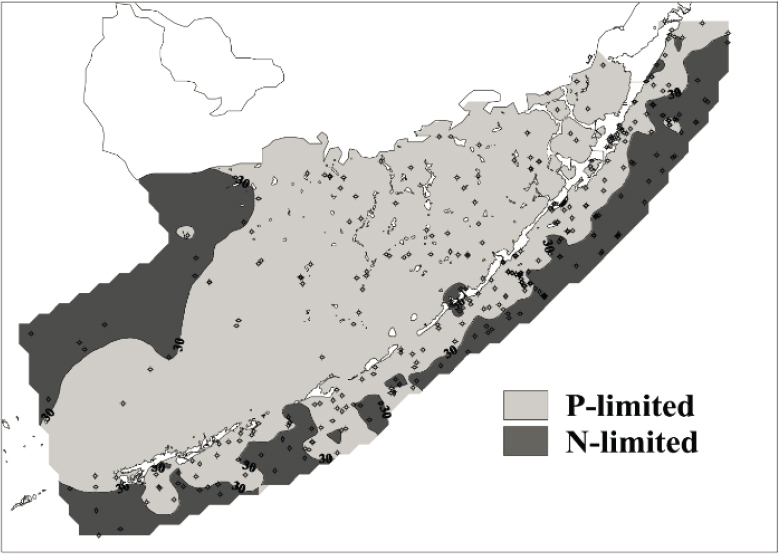
SOURCE: Fourqurean and Zieman, 2002. Reprinted with permission; copyright 2002, Springer Nature.
inputs to the bay. Trends in phosphorus concentrations in Florida Bay over time appear relatively steady, with the exception of large hurricane events, which can elevate total phosphorus concentrations for up to 2 years afterward (Cole et al., 2018). Significant excursions of phosphate concentrations have also been observed after seagrass die-off events; Fredley et al. (2019) observed phosphate concentrations several times greater than pre-die-off levels in affected basins.
Following the 1987-1994 event, more than a decade of research ultimately uncovered a suite of interconnected contributing factors: hypersalinity caused by low freshwater inflows combined with high temperatures in areas with dense seagrass beds (Carlson et al., 2018; Fourqurean and Roblee, 1999; Johnson et al., 2018; McIvor et al., 1994; Zieman et al., 1999). These conditions triggered a cascade of biogeochemical and ecological effects. Together with high temperatures, the hypersaline conditions caused hypoxic conditions and high levels of sulfide,
which is lethal to plants (Koch et al., 2007; Rudnick et al., 2005), resulting in widespread seagrass die-off in the central and western portions of the Bay. The process is outlined in more detail in Figure 5-22. This series of events represents a collapse at the base of the food web, and the consequences included unstable sediments, algal blooms, and increased turbidity (Deis, 2011; Hall et al., 1999), with major effects on commercial and recreational fishing. Although the system recovered over a 20-year period, there have been environmental concerns since, including a second seagrass die-off in 2015; both events were similar in scope of near total mortality (over 90 km2) and location (Figure 5-21). Ultimately, more than 160 km2 were affected in 2015, and the cascade of ecological responses
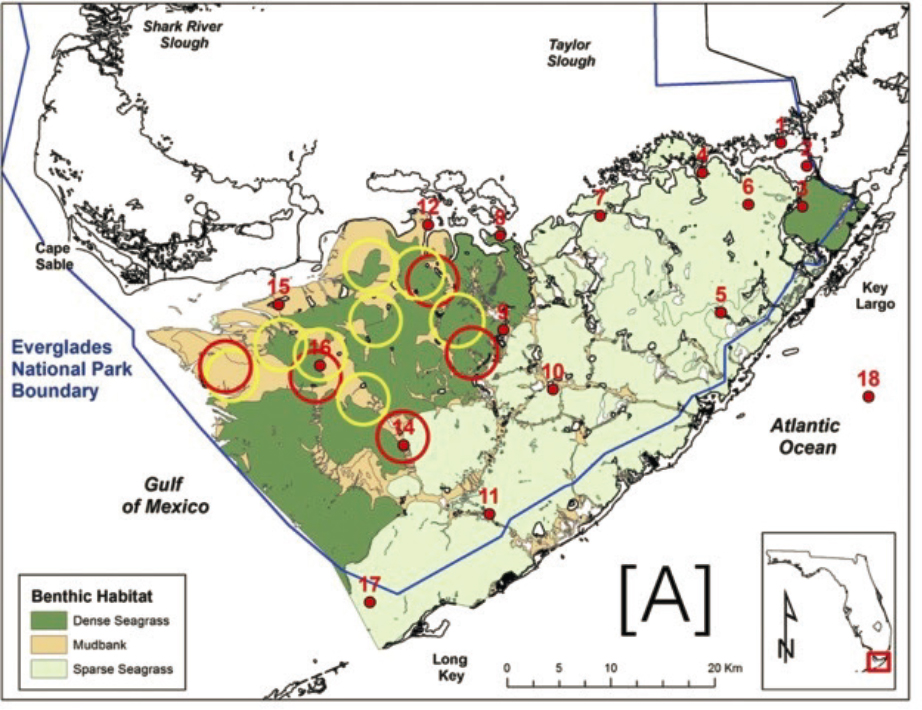
SOURCE: Carlson et al., 2018.
followed similar conditions as that in the 1980s—localized drought, high temperatures, and hypersalinity.
Ecological implications.
Two large seagrass die-off events in recent history (beginning in 1987 and 2015) caused substantial ecological degradation, including widespread turtlegrass (Thalassia testudinum) mortality (Figure 5-22). The first die-off was notable in multiple ways: the speed of weeks to months, affecting Thalassia only at a large spatial scale, with small-scale patchiness, reticulated distribution, and sharp transitional boundaries (Hall et al., 2016). A multiyear
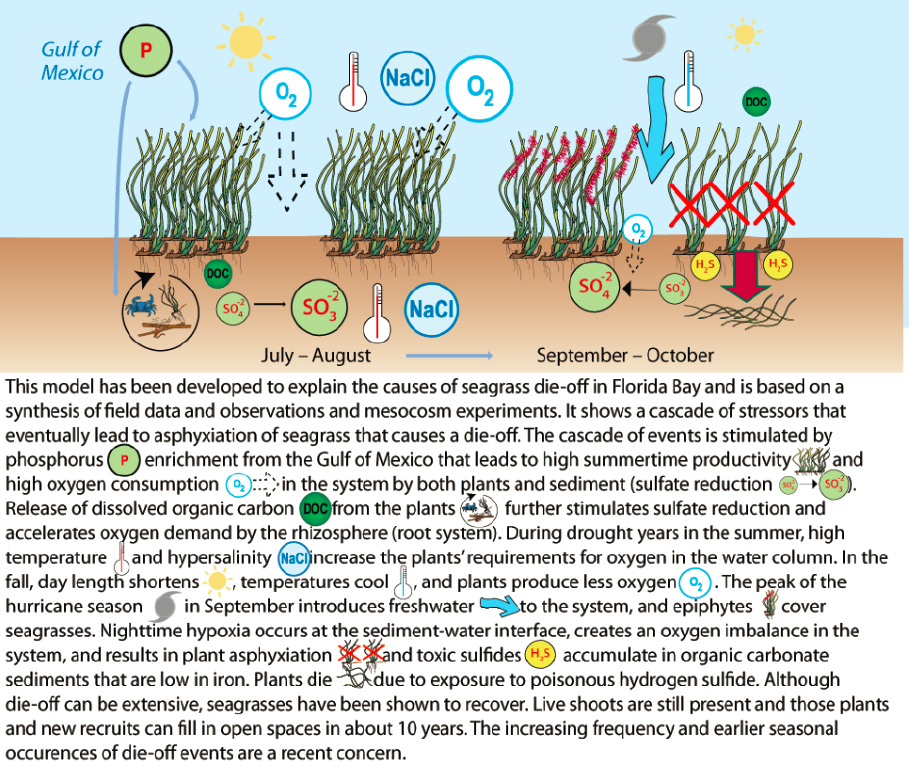
SOURCE: Kruzynski and Fletcher, 2012. Reprinted with permission; copyright 2012, Ian Press/University of Maryland Center for Environmental Science.
drought followed this event, and a cascade of ecological effects was documented. Widespread and persistent blooms of cyanobacteria (Synechococcus sp.; Berry et al., 2015; Glibert et al., 2009; Phlips et al., 1999) and widespread turbidity due to resuspended sediments coincided with large-scale decimation of sponge communities, causing loss of critical nursery habitat for important commercial species, including spiny lobster (Panuliris argus) and pink shrimp (Penaesus duoranum) (Butler et al., 1995; Fourqurean and Robblee, 1999; Peterson et al., 2006; Robblee et al., 1991).
Both events originated in the northern portions of the Central Florida Bay. Cole et al. (2018) found that basins with severe seagrass die-off in 2015, when sampled more than 2 years before, had higher total phosphorus concentrations and seagrass cover (dense, monotypic stands of Thalassia) than basins that did not experience severe die-off. Basins with severe seagrass die-off also had lower dissolved inorganic nitrogen (DIN) and a lower ratio of DIN to phosphorus, indicating that they were less phosphorus limited. These findings suggest the need for increased freshwater flows in the northern central regions of the Bay (e.g., Rankin Bight)—areas with dense seagrass supported by marine phosphorus inputs—to prevent hypersalinity events and reduce the frequency of future seagrass die-off events.
Improved volumes and timing of freshwater flows could also enhance the diversity of seagrass species, which could help strengthen the resilience of the Bay to future seagrass die-off events (Herbert et al., 2011). Seagrasses are sensitive to salinity. Changes in salinity regimes over the past several decades are thought to have contributed to a shift in seagrass species distributions (Fourqurean et al., 2003; Zieman, 1982). Halodule wrightii, a species that favors lower mean salinities and more variable salinity conditions than Thalassia, was the observed dominant species in much of north and northeastern Florida Bay prior to the 1970s, while Thalassia typically dominated the western part of the bay (Zieman et al., 1989). By the time of the late 1980s die-off event, north and northeastern Florida Bay were dominated by high-density, monotypic stands of Thalassia, which are considered the most vulnerable to die-off events (Hall et al., 2016). Return to a mixed species composition may also support higher fish densities (Chester and Thayer, 1990; Thayer et al., 1999).
Restoration Goals and Expected Near-Term Effects of CERP Projects
In light of the seagrass die-off in the late 1980s and early 1990s (Zieman et al., 1999), the CERP aimed to increase overland flow into Everglades National Park and Florida Bay to improve salinity conditions. The broad CERP goals related to Florida Bay are captured in the 2007 Interim Goals Agreement (USACE et al., 2007):
- Increase freshwater flows to Florida Bay;
- Reduce the intensity, duration, frequency, and spatial extent of high-salinity events, reestablish low-salinity conditions in mainland nearshore areas, and reduce the frequency of and rapidity of salinity fluctuations resulting from pulse releases of freshwater from canals;
- Minimize the magnitude, duration, and spatial extent of algal blooms in Florida Bay;
- Reestablish a diverse seagrass community with moderate plant densities and more natural seasonality, and increase the percentage of Florida Bay having suitable habitat for seagrass growth;
- Increase densities of juvenile shrimp within the various basins of Florida Bay; and
- Increase the frequency of salinities less than 20 ppt in the northern enclosed sub-basins of Florida Bay to foster optimal growth and survival of juvenile crocodiles
Yellow Book projections for Florida Bay.
Collectively, CERP projects, including new southern sources of water storage in the Lake Belt, were expected to “improve the salinity regime in the coastal basins adjacent to Florida Bay” (FWS, 1999). However, scientists and planners did not have reliable flow estimates from the CERP to Florida Bay, due to the coarse (2 mile x 2 mile) grid size used by the South Florida Water Management Model and the difficulty of accurately simulating flows near the coasts. Since the CERP was launched, extensive research has led to a broad scientific consensus that wetter conditions prevailed in the historic system than previously thought (McVoy et al., 2011; NASEM, 2016). Restoring predrainage conditions in the Everglades and Florida Bay would require substantially greater water depths, flow volumes, and flow velocities than assumed in the Yellow Book (Marshall et al., 2020; RECOVER, 2011a).
Expected effects of planned CERP projects.
Two currently authorized CERP projects—CEPP (including the EAA Reservoir) and the C-111 Spreader Canal (Western) Project—affect Florida Bay. Modeling for the RECOVER Interim Goals analysis shows that, collectively, these projects are expected to increase surface-water flows to Florida Bay (across the T23 transect [see Figure 5-23]) during the dry season by 50 percent (or 28,000 AF) (RECOVER, 2020b). During the wet season, flows are slightly reduced (by 3,000 AF). Although this is not a large increase in average flow on an annual basis (11 percent) and far below predrainage flows, these discharges are expected to reduce salinity in nearshore basins, particularly in North Florida Bay (Figure 5-19). This increase in flows is expected to lead to a decrease in annual mean salinity of 1.4 and 2.8 ppt in Little Madeira Bay and Terrapin Bay, respectively, with comparable reductions in 75th percentile salinities.
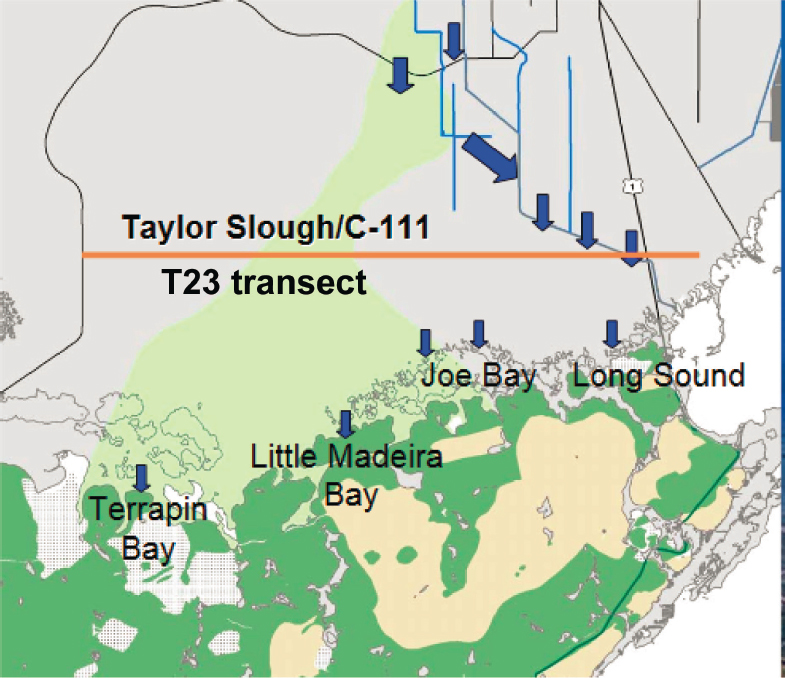
SOURCE: DOI, 2009.
USACE and SFWMD (2014) noted that the CEPP represented a 12 percent improvement toward the full restoration salinity target (Figure 5-24). The addition of the EAA reservoir to the CEPP showed relatively minor effects on Florida Bay, with an additional 7,000 AF per year above the CEPP, with a corresponding limited decrease in salinity of 0.05 PSU (USACE, 2020a). Part of the reason such little benefit is seen from the EAA Reservoir is that equivalent seepage management was not included in the project to facilitate conveyance to the south without exceeding flood constraints. Southern Everglades is a future CERP planning initiative that will include several project components from the Yellow Book that could benefit Florida Bay if implemented, including Everglades National Park (ENP) Seepage Management, Lake Belt Storage, and Lake Okeechobee ASR. The planning process for southern Everglades is scheduled to begin in 2023 (USACE, 2020c).
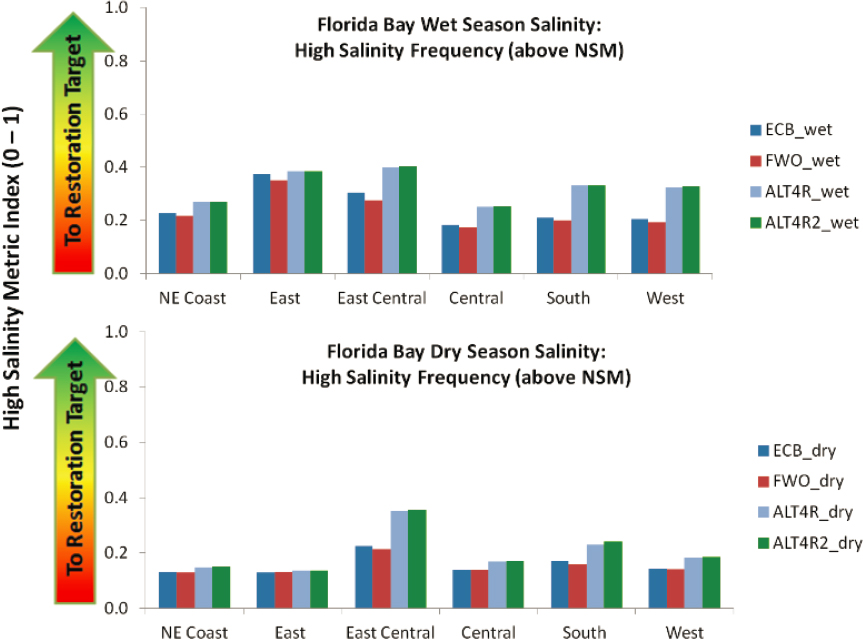
SOURCE: USACE and SFWMD, 2014.
As discussed in Chapter 4, the Combined Operational Plan (not included in the Interim Goals modeling) is projected to increase freshwater flows into Florida Bay by 36,000 AF per year, which is greater than that projected from the CEPP and the EAA Reservoir. The greatest increases in overland flow occur through the Eastern Panhandle to East Florida Bay.
Implications of Environmental Issues for CERP and Non-CERP Efforts
The CEPP and the C-111 Spreader Canal (Western) Project provide most of the Florida Bay salinity benefits to the East Central Florida Bay (Figure 5-24)
through increases in Taylor Slough flows. The COP also provides most of its benefits to East and East Central Florida Bay. The benefits, however, are relatively modest compared to predrainage flows. Restoring predrainage flow to Florida Bay is not a realistic expectation given the massive reduction in natural water storage in the Everglades ecosystem, and therefore it is even more important to understand the spatially explicit benefits to Florida Bay of the water that is delivered. Given the size of Florida Bay and its restricted circulation, increased CERP flows to only the eastern and east-central portions of the Bay will likely have limited effects on salinity on Central and West Florida Bay. Yet, the two notable seagrass die-off events started in the Central Bay (Figure 5-21), where dense seagrass beds exist and mud banks significantly limit circulation—conditions which are essential to a die-off event. Additional efforts beyond the current authorized projects, either within or outside of the CERP, would be needed to meet CERP’s goals for Florida Bay related to hypersalinity events, seagrass, and algal blooms.
Key Questions to Inform Management Decisions in Florida Bay
Much research has been done to investigate the role of freshwater flow in the environmental problems of Florida Bay and to utilize a combination of monitoring and modeling to quantify how CERP projects will impact freshwater delivery relative to flow and salinity targets. However, Florida Bay is influenced not only by this freshwater inflow and its inherent water quality but also by the Gulf of Mexico; both hydrologic drivers will change in the future as a function of global land use and climate change. Given the size of public investment in restoration, as well as the emergence of new challenges since the CERP was first envisioned, adaptive management of the CERP will be critical to meeting restoration goals for Florida Bay. This requires the identification of key questions central to addressing problems that need to be addressed to develop appropriate management responses. Examples of questions relevant to Florida Bay management decisions are provided in Box 5-8.
Science Support for Restoration Management Decisions in Florida Bay
A long history of research has led to a robust understanding of the changing ecological status of Florida Bay and its causes. However, current restoration efforts are proceeding under conditions that are changing rapidly toward a future with uncertainty, and a new set of tools is necessary. Monitoring and research, syntheses, and modeling could be used to address the key questions posed above under these new conditions; these new tools would include a refined set of ecological expectations, quantitative understanding of spatial trade-offs between
various flow management approaches, methods to operationalize decisions, and support of long-term regional planning and coordinated management actions among CERP and non-CERP efforts. The status of these tools, as they apply specifically to Florida Bay, are reviewed in the following sections.
Biological effects monitoring, synthesis, and modeling.
Florida Bay has been the focus of a range of scientific inquiry efforts by both agency and academic personnel, with remarkable progress via causal investigations linking biological
outcomes, such as seagrass communities and alligators, to environmental drivers, principally freshwater flow. Multidisciplinary investigations of the drivers and causal relationships behind the first massive seagrass die-off in Florida Bay is an excellent example of a living science model, where two decades of monitoring, experiments, and modeling research supported by Alligator Alley toll funds, RECOVER, and competitive federal grants in combination with the multiagency Marine Monitoring Network iteratively identified the interplay of five causal factors contributing to this die-off and subsequent recovery trajectories: upstream discharge, hypersalinity, high water temperatures, low wind speeds, and low dissolved oxygen (Figure 5-22; Johnson et al., 2018; Rudnick et al., 2005; Zieman et al., 1999). It was also recognized that the shift in seagrass community composition since the 1960s—from mixed species stands to Thalassia-dominated, dense, monotypic stands (Fourqurean et al., 2003; Zieman, 1982)—increased vulnerability to the initiation of die-off events.
This level of understanding allowed the development of two ecological models that link salinity and other drivers to seagrass die-off and community structure: the Florida Bay Seagrass Community Model (SEACOM; Madden and McDonald, 2006, 2007) and a statistical discriminant function model that assigns a probability of eight seagrass community types occurring for a given combination of water quality variables (Fourqurean et al., 2003; Herbert et al., 2011). While SEACOM is a physiological/mechanistic model (one that attempts to represent the interaction of multiple physical, chemical, and biological processes in real time), the second is a statistical model (based on demonstrated relationships between a suite of water quality parameters and the occurrence of various seagrass communities). The existence of both provides robustness to our understanding of seagrass community processes and represents an advanced capability for the type of ecological forecasting that is necessary for adaptive management of the restoration effort.
SEACOM is a process-based seagrass biomass unit model that was developed to capture the effects of freshwater inflows and hypersalinity, sea level, high water temperatures, low wind speeds, and low dissolved oxygen, which drive the production of sediment sulfides that are toxic to seagrass plants (Johnson et al., 2018). The model was developed to evaluate these mechanisms and their effects on seagrass community processes, distribution, and survival (Madden et al., 2016), thereby informing and improving coastal management of seagrass systems. The model currently includes fully integrated Halodule wrightii and Syringodium filiforme modules in addition to Thalassia, and a provisional Ruppia maritima module, calibrated for nine basins that represent a large part of Florida Bay. SEACOM has been used in a number of management decisions, utilizing the hydrology and water quality variables produced by another model-
ing effort, FATHOM, as input. Thus, SEACOM results could only be as spatially explicit as FATHOM results (54 basins covering Florida Bay). SEACOM model code will eventually be incorporated into a 3D regional ocean modeling system hydrodynamic/water quality model that will enable full spatially explicit simulation of seagrass dynamics across the Bay and at the sub-basin scale (C. Madden, SFWMD, personal communication, 2020).
A second seagrass ecological model links environmental drivers to seagrass species composition. Because seagrass species exhibit different sensitivities to salinity climates, models are available to predict potential shifts in seagrass communities with changes in freshwater flow expected under the CERP. Herbert et al. (2011) used salinity climates from the hydrodynamic model FATHOM as input to a statistical discriminant function model that associates eight seagrass community types with water quality variables including salinity, salinity variability, total organic carbon, total phosphorus, nitrate, and ammonium, as well as sediment depth and light reaching the benthos. This ecological forecasting is valuable in setting expectations for the restoration effort. Salinity climates in the western sub-basins were expectedly insensitive to modeled increases in freshwater inflow, while the north, northeastern, and eastern sub-basins were highly sensitive and exhibited predicted and favorable shifts in community composition (Herbert et al., 2011). It is notable that this second model also utilizes FATHOM as input, and thereby is limited in its ability to predict at a finer spatial scale. Thus, both seagrass models cannot be made spatially explicit without a hydrodynamic model that provides higher-resolution predictions than are currently available with FATHOM.
Florida Bay monitoring and research have also supported the development of other ecological models. A crocodile habitat suitability index, which characterizes the suitable habitat, based on observed salinities, nest sites, and prey biomass during periods in which hatchlings are the most vulnerable to high salinities (Brandt, 2013), was utilized to assess CEPP alternatives. A spatially explicit, stage-based population model has been developed and run for assessment of the CEPP (Green et al., 2014). A habitat suitability model is also available for pink shrimp (Mulholland, 1984), and pink shrimp have been advocated as a performance metric for Florida Bay restoration (Browder and Robblee, 2009), although neither are currently used in restoration planning.
The tipping points associated with seagrass die-off events involve more than salinity. There was high spatial coincidence between the Thalassia die-off events initiated in 2015 and 1987, and hypersalinity, water column stratification, and bottom-water anoxia were again identified as major causal factors (Hall et al., 2016). Although the basic conceptual model is accepted, the climatic conditions that precipitate hypoxic stress within the densest meadows is still unclear
and may involve a combination of several meteorological events that lead to increased evaporation or stratification in these precise areas. Conditions for recovery are even less understood; although almost full recovery from the 1987-1990 die-off occurred over the ensuing 20 years and followed paradigms of Thalassia succession (Hall et al., 2016), the conditions that supported it have not been characterized or summarized. Seagrass monitoring (Madden et al., 2009; RECOVER, 2007a) could be useful as early warning signals of vulnerability to die-off events as well as indicator conditions necessary for sustained recovery, allowing for improved operational decision making.
Hydrologic and hydrodynamic modeling and observations.
The ability of ecological models to provide spatially and temporally explicit information to support decision making is constrained by the corresponding resolution of the data for the relevant environmental drivers. Direct and quantitative linkage of Florida Bay salinities—important forcing terms for seagrass and other ecological models—to southern Everglades freshwater deliveries has been problematic. Salinity is affected by upstream flows, evapotranspiration, precipitation, and circulation, and, therefore, spatially explicit salinity predictions require accurate models of surface- and groundwater inflows and bay hydrodynamics. Especially challenging is the ability to simulate surface-and groundwater flows at the coastal boundary and their effects on the salinity of the North and northern Central Bay, where the CERP is expected to have the greatest potential impact.
Florida Bay hydrodynamic models (FATHOM and EFDC) and water salinity models (FATHOM) have been developed and validated by management agencies to support restoration conversations. However, these management-adopted models have not been fully integrated with upstream hydrologic drivers, and further model development and integration efforts are needed. Additionally, significant data gaps, such as uncertainty about the role of groundwater flow as a driver of Florida Bay response, require further investigation. Currently, in the absence of more explicit tools, CERP planning has relied on the use of multiple linear regression models (Marshall et al., 2011), which relate inland hydrologic variables (e.g., stage) and marine and weather data to predict salinity at 37 Florida Bay index areas. The models reproduce daily salinity simulations that are capable of estimating 65 to 80 percent of the daily variability in salinity depending upon the model; the models have little bias, but the absolute daily error in the nearshore embayments and the mangrove zone can be sizable during seasonal transitions on particular daily simulation. FATHOM in combination with multiple linear regression models to date has successfully supported management conversations on minimum flow (SFWMD, 2006, 2014) and CERP restoration planning discussions (USACE and SFWMD, 2014). EFDC is a 3D finite difference hydrodynamic model that was developed for Florida Bay through successive iterations in 2004
and 2008. EFDC was originally intended to be coupled to a water quality model to simulate factors that could affect seagrass die-off. The model development went through model validation stages but reportedly did not progress because of long run times and significant issues with the water quality module (Hamrick and Li, 2008). The original vision for the EFDC hydrodynamic model was to couple it with a biogeochemical submodel and link these to a coastal hydrodynamic model (SF-HYCOM; see Table 5-7) to provide Gulf of Mexico boundary conditions for the EFDC model, which would provide a mechanism to explore the effects of climate change, although these linkages were never completed.
Spatially explicit water and salinity budgets of Florida Bay have been challenging to construct, however, because the magnitudes, seasonality, and spatial distributions of southern Everglades inflows are poorly understood (Mills et al., 2019). Models that link the hydrodynamics of estuarine and coastal waters with coastal wetlands and watersheds remain insufficient to address fine-scale water distribution issues, groundwater–surface water exchange, saltwater intrusion, and the resulting effects on plant communities, soils, sediments, and biogeochemical cycles. Mills et al. (2019) noted that accurate predictions of salinity in northern Florida Bay requires moving beyond static stage-salinity relationships (Marshall et al., 2011) to linked hydrologic and hydrodynamic models. As discussed previously (see Biscayne Bay), the BISECT model (Swain et al., 2019) is designed to connect regional hydrologic models (e.g., RSM-GL) with hydrodynamic models using three-dimensional density-dependent flow modeling. BISECT (Swain et al., 2019) has been already used to begin investigating sea-level rise scenarios on groundwater and surface-water flows and saltwater intrusion. As in Biscayne Bay, however, the accuracy of the model is limited by the lack of monitoring data in some regions. Mills et al. (2019) note that “to properly calibrate such a model, a network of existing monitoring wells may need to be improved/validated and additional new groundwater wells may need to be strategically installed in ENP [Everglades National Park] to assess surface water, ground water and surficial water flows and volumes from ENP into the Bay.”
In addition, there is a need for comprehensive scenario assessment modeling to support operational actions that could increase freshwater flow, especially into northern Central Florida Bay, where algal blooms have historically originated. For example, questions exist regarding the ability to increase surface-water flow and seepage through Buttonwood Ridge into northern portions of Central Florida Bay, which may require operations to convey water to western Taylor Slough, potentially when Shark Slough stages are high enough to overcome barriers to flow. Assessment of how much water can be recovered from seepage at the eastern boundary of Everglades National Park is also an outstanding issue that could be addressed with additional groundwater modeling.
Summary: Integrated Modeling for the Southern Coastal Systems
In the long term, an integrated modeling framework of coastal response for the entirety of southern Florida that describes the interaction of human activities and ecological response is desirable to guide restoration investments and inform evaluations of trade-offs. Such models are necessary to examine conditions that trigger tipping points for seagrass die-off events and enhance the recovery from those events. Because of the complex number of factors that have to co-occur both spatially and temporally (e.g., nutrients and chlorophyll concentrations, salinity, temperature, circulation, stratification), integrated monitoring and modeling is critical to identify actions that are necessary and appropriate to avoid these tipping points. Project and operations decisions also inherently weigh differential benefits for ecological regions (e.g., Shark Slough versus Taylor Slough, Biscayne Bay versus Florida Bay) and balance a range of objectives (e.g., restoration, flood control, water supply). Integrated modeling along the southern coastal systems can provide consistent information to clarify the trade-offs between alternatives at a systems scale and improve decision making.
The effects of sea-level rise, ocean warming, and acidification are anticipated to increase over the next 50 years, further increasing the needs for spatially explicit, mechanistic hydrodynamic and biogeochemical models, coupled to biological models to inform CERP implementation and adaptive management. August maximum sea surface temperature has already increased by 1°C between 1985 and 2016, making the future viability of seagrass uncertain (Carlson et al., 2018). Circulation and nutrient supply from the Gulf will change with sea-level rise, requiring new or improved models for salinity and water quality prediction and cascading effects. For example, declining pH from ocean acidification can dissolve carbonate sediments with associated biogenic phosphorus, increasing phosphorus availability and seagrass biomass (Jensen et al., 2009), while increased warming and hypoxia can make high-biomass seagrass beds more susceptible to die-off (Carlson et al., 2018). Brackish and freshwater peat marshes are at risk of subsidence with saltwater intrusion, leading to an associated release of nutrients (Wilson et al., 2018); the importance and complexity of the response of this portion of the coastal landscape to sea-level rise was covered in NASEM (2018).
Changes in water management may be necessary to maintain the elevation of these coastal wetlands and prevent peat collapse conversion to open water (Mills et al., 2019). Integrated modeling tools can be used to examine strategies that increase hydroperiods in coastal and near-coastal wetlands and reduce the effects of saltwater intrusion or increase surface-water flows to areas at the greatest risk of seagrass die-offs. While South Florida hydrologic observations, synthesis, and modeling are relatively advanced, this toolkit has not been used
TABLE 5-9 Criteria and Status of Monitoring, Research, Syntheses and Predictive Modeling Tools for the Southern Estuaries
Category | Criteria for Status of Scientific Components | Status | |
---|---|---|---|
Florida Bay | Biscayne Bay | ||
Integrated Hydrologic System Modeling | Terrestrial hydrologic basin predictions can capture links in the hydrologic cycle from northern everglades, WCAs, STAs and water resource operations (including impacts of climate change) | Advanced | Advanced |
Coupled freshwater–groundwater predications to estuaries and estuary hydrodynamic models: freshwater flows from surface and groundwater, ocean tidal forcing and water surface elevation, estuarine hydrodynamic water, salinity | Intermediate | Intermediate | |
Watershed and Estuarine Water Quality | Watershed water quality can simulate (as a function of external and internal drivers) temperature, total nitrogen and total phosphorus, dissolved organic and inorganic nutrients, and chlorophyll a | Early stage | Early stage |
Estuarine water quality: Ability to estimate/model salinity and mass balances of nutrients as a result of ocean and freshwater mixing, model the combined extinction of light from turbidity and chlorophyll a (primary productivity) | Intermediate | Early stage | |
Biological Models | Seagrass: Can predict physiological effects of temperature, salinity, light limitation (turbidity, water color and chlorophyll a), and substrate on seagrass biomass | Advanced | Intermediate |
Fauna: Spatially explicit models that provide quantitative estimates of habitat to target species and remediate local stressors within sub-basins or sections of the Bays | NA | Early stage |
NOTES: Status ranges from emerging (observation or science components not yet implemented to capture relationships between drivers and environmental problems), early stage (basic relationships observed and reported but data gaps that inhibit formulation of advanced predictive tools have not yet been addressed), intermediate (observations and research synthesized and preliminary predictive tools developed, more developments/refinements are needed), advanced (predictive tools validated and in routine use). STA, stormwater treatment area; WCA, Water Conservation Area.
to its full capacity to investigate the effects of climate change and its impacts on the human and natural ecosystem components of the Greater Everglades. A summary of the status of readiness of the toolkit is provided in Table 5-9.
CONCLUSIONS AND RECOMMENDATIONS
The CERP will help address freshwater inflow concerns in all of the estuaries but it is only part of the solution. CERP ecological restoration goals, particularly in the northern estuaries and Biscayne Bay, cannot be met if water quality and associated algal blooms, which are outside of the direct purview
of the CERP, are not addressed. CERP projects primarily aim to improve hydrologic and ecological conditions in the estuaries by enhancing the volume and timing of freshwater inflows, thereby bettering salinity conditions. However, additional hydrologic restoration beyond those planned to date for the CERP may be needed to meet stakeholder expectations for estuary recovery (e.g., reducing high-volume flows derived from local watersheds in the northern estuaries). Some CERP projects are expected to reduce nutrient loads, but the water quality components of CERP projects represent only minor aspects of the steps needed to meet water quality criteria in the estuaries. Requirements for compliance with the Clean Water Act to address pollution and water quality fall to the state and not to the CERP. Public expectations for improved estuarine conditions, such as healthy seagrass meadows, improved oyster habitat, and control of harmful algal blooms, extend beyond what the CERP alone can achieve and require both CERP actions and water quality and habitat improvements through non-CERP efforts. CERP planning has not rigorously considered the potential impacts of impaired water quality on its ecological goals. Understanding the collective impacts of hydrology and water quality in meeting restoration goals and stakeholder expectations is essential to support ongoing CERP and non-CERP management decisions. If the impacts of water quality are not well understood, CERP water management projects may be unfairly blamed for failing to meet expected outcomes.
CERP goals for the southern estuaries should be revisited and clarified in light of improved ecosystem understanding and modeling capabilities. Early formulations of the CERP had qualitative objectives for Biscayne and Florida Bays. Freshwater flow targets linked to spatially specific ecological goals were never developed for use in CERP planning because predrainage flows were not well understood and model predictions were poor along the coastal boundaries. For example, in Biscayne Bay, nearshore salinity goals were developed, but the absence of freshwater flow targets complicates an understanding of what is attainable. In Florida Bay, the authorized CERP and non-CERP projects (including the CEPP and the COP) do little to address the specific region where historic seagrass die-offs occurred. Analysis of ways to optimize CERP outcomes with available flows requires more spatially targeted goals for the region. Analysis of what can be achieved through the CERP is essential to manage stakeholder expectations and, if appropriate, motivate additional non-CERP efforts. Additionally, these analyses will facilitate evaluations of trade-offs in water use among other water users and other regions of the ecosystem.
Existing data and tools should be used to improve science support for decision making across the estuaries. The relevant agencies have a long history of monitoring, but existing modeling tools and data sets are underutilized. Models
and monitoring data offer opportunities to rigorously examine restoration alternatives and constraints, better understand trade-offs, and develop management strategies to enhance restoration benefits.
CERP and non-CERP agencies will need an advanced set of predictive tools, developed and implemented through effective coordination among scientists and managers, to better support critical water management decisions ahead. High-priority science and modeling needs include
- Spatially explicit water quality models and a sustained program of observation and research to build toward a predictive harmful algal bloom modeling toolkit for the northern estuaries;
- Watershed loading and water quality models to predict effects of salinity, water quality, and light limitation on the viability of seagrass in Biscayne Bay;
- Spatially explicit and mechanistic biological models (e.g., seagrass, oyster), developed from appropriately scaled and sustained monitoring programs for the northern estuaries that can capture the quantitative basis for relationships between freshwater flows, water quality drivers, and biological outcomes of interest;
- Predictive tools to identify thresholds and tipping points in all the estuaries, such as the complex factors associated with algal blooms and seagrass die-off;
- A southern Everglades transition-zone observational and modeling program that supports project planning and can couple regional hydrologic models, including groundwater–surface water exchange, with spatially explicit estuarine hydrodynamic and salinity models; and
- Integration of modeling and observations across the entire southern inland and coastal system to evaluate cross-project synergies and ecological responses (e.g., the ecological response of Biscayne Bay and Florida Bay to enhanced seepage management).
Clarity in critical future water management decisions can help prioritize additional research, monitoring, modeling, and synthesis efforts to better support CERP and non-CERP initiatives. Open communication and cooperation between subregion research, observational, and model development teams are needed to facilitate improved model coupling, accelerate knowledge gains, and allow models to collectively address trade-off decisions. Advancement in modeling could benefit from improved coordination across the estuaries to accelerate knowledge gains and allow broader regional approaches to address trade-offs in decisions.
Climate change and sea-level rise will have major effects on the estuaries, and those effects need to be better understood to inform management decisions and develop strategies that will provide long-term restoration benefits.
Terrestrial hydrologic monitoring, synthesis, and modeling in South Florida are relatively advanced, but this toolkit has not been applied to investigate the effects of climate change on the human and natural systems of the South Florida Everglades and associated estuaries. In the northern estuaries, estuarine hydrodynamic modeling is advanced, but in Florida Bay and Biscayne Bay, improvements to these modeling capabilities are needed. Improved modeling of coastal boundaries is required to understand the implications of sea-level rise on groundwater and surface-water inflows and saltwater intrusion. Additional research is needed to extend these climate scenario predictions from effects on hydrology to effects on water quality and ecosystems, such as the accompanying effects of saltwater intrusion on peat loss and subsidence. To ready the toolkit for this exercise, investments recommended above to make water quality and biological models increasingly mechanistic and spatially explicit will also serve to credibly predict impacts from climate change stressors. This information can then be used to examine the long-term performance of projects and identify possible adaptive management strategies or design alterations to increase ecosystem resilience.