4
Effectiveness of Nuclear Weapons Against Hard and Deeply Buried Targets
TARGET DESTRUCTION
The types of hard and deeply buried targets (HDBTs) of interest are described in Chapter 2. These range from hardened, surface bunker complexes to tunnel complexes deep underground, as shown in Figure 2.1.
Nuclear weapons are the only weapons that can destroy targets deep underground or in tunnels. Weapons of mass destruction (WMD)-related facilities near the surface may be destroyed with either nuclear or conventional weapons, but nuclear weapons are of interest because they produce more effective agent-kill mechanisms. The types of conventional weapons likely to be employed against tactical targets are discussed in Chapter 7, “Conventional Weapons.” Note that the discussion in this chapter assumes the physical destruction of the target. It may also be possible to destroy or degrade the functionality of the facility, or the functionality of the network of which it is a part, without physically destroying the specific target node.
The following are elements of target destruction:
-
Finding, identifying, and characterizing the target;
-
Weapon-system survival and arrival at the target;
-
Weapon penetration and detonation;
-
Energy coupling of weapons effects to the ground;
-
Shock propagation through the ground to the target facility; and
-
Response and vulnerability of the target facility.
Finding, Identifying, and Characterizing the Target
This report examines the relative effectiveness of and collateral damage associated with nuclear earth-penetrator and other weapons. It is not within the scope of the committee’s tasks to analyze the
quality of the intelligence that exists or will exist. Nor does the committee discuss how good the intelligence must be to enable the effective operational use of a weapon.
The discussions and calculations presented in this report assume knowledge of the target’s location, purpose, size, function, internal layout, and other relevant features at the time it may be attacked. This assumption of perfect, timely intelligence is unlikely to hold in reality for the vast majority of targets of interest.
Important to the issue of finding, identifying, and characterizing a target is that, in addition to concealment, deceptive techniques are used extensively by adversaries to complicate matters. The calculations presented should all be viewed with these intelligence uncertainties in mind.
Weapon-System Survival and Arrival at the Target
In addition to the passive defense of hardening a target, high-value sites are generally defended. Thus, the method of delivery of a weapon is important. For example, defenses against a weapon that is air-delivered are quite widespread, whereas ballistic missile defenses are virtually nonexistent. This report does not examine the effectiveness of delivery modes or defenses against them.
Weapon Penetration and Detonation
There is the need to consider the probability that an earth-penetrator weapon (EPW) will survive ground penetration, penetrate to the desired depth, and then successfully detonate. In comparison with surface (contact) burst weapons, the EPW experiences more rigorous impact conditions. These factors are discussed in Chapter 3.
In the following discussion of weapons effects at depth, the committee presumes that the nuclear earth-penetrator weapon successfully reaches the target, penetrates any aboveground covering structure and possible defenses, and enters the surface to a depth sufficient to couple the majority of its energy to the ground, all with a probability of 1.0. The committee did not study the probability of any of these events.
Energy Coupling of Nuclear Weapons Effects to the Ground
The energy coupled by a nuclear weapon to the ground is expressed as the fraction of the total weapon yield converted to kinetic energy of downward-moving solid or nonvaporized ground material. The amount of energy coupled to the ground is strongly dependent on the weapon’s actual height of burst (HOB) or depth of burst (DOB), as well as on nuclear design details (i.e., yield-to-mass ratio, fission fractions, and relative coupling efficiencies of the source components). Geologic properties also play a role.
Effects Manual-1: Capabilities of Nuclear Weapons1 of the Defense Threat Reduction Agency (DTRA) (formerly the Defense Nuclear Agency) defines an equivalent yield factor for both total coupled energy and ground-shock-coupled energy as a function of HOB/DOB (see Appendix C in this report for details). Figure 4.1 shows the equivalent yield factors normalized to a contact burst using the DTRA-recommended, scaled HOB of 0.05 m/kt1/3. Note that the coupled energy is not defined for a scaled HOB greater than −0.05 m/ktl/3 or a scaled DOB greater than 0.05 m/kt1/3 due to uncertainties in calculations for this near-surface region. The ground-shock-coupled energy2 includes surface air-blast-induced ground shock.
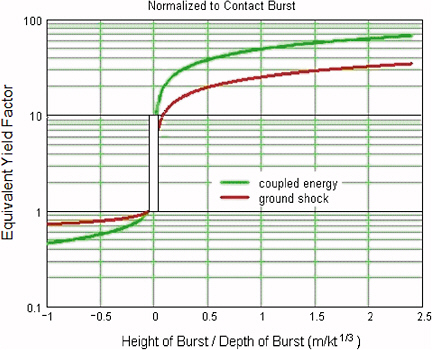
FIGURE 4.1 Equivalent yield factors for total coupled energy and ground-shock-coupled energy normalized to a contact burst. Positive numbers signify below ground. (See Appendix C.)
Equivalent yield factors for coupled energy asymptotically approach 100 (i.e., as burial depth increases); indeed an earth-penetrator weapon is effectively fully coupled at a scaled DOB of about 2.3 m/kt1/3. Relative to a contact burst, the ground-shock-coupling factor approaches 50 with increased DOB owing to the surface air-blast contribution.3
For a generic 300 kiloton EPW at 3 meters depth of burst (scaled DOB = 3/(300)1/3 = 0.45), the ground-shock-coupling factor is about 20, which is equivalent to a contact burst of about 6.0 megatons.4 This example illustrates the “efficiency” of an earth-penetrator nuclear weapon in generating comparable levels of damaging ground shock at target depth with significantly lower yield relative to a surface-burst or airburst weapon. As mentioned elsewhere, the coupling factor can be anywhere between 15 and 25, with the greatest uncertainty due to the effect of the radiation from a surface burst, which is sensitive to local conditions.
Uncertainties in Weapon Effectiveness, Energy Coupling, and Ground-Shock Range to Effect
The following factors influence the uncertainties associated with estimating energy coupling for ground shock.5
-
Weapon design. Energy deposition into the ground involves two components, which couple differently: debris kinetic energy and x-rays. The physical dimensions and the location of the primary component relative to the secondary also affect energy deposition.
-
Actual height of burst (HOB) or depth of burst (DOB). Coupling increases by more than an order of magnitude in the region between contact and 1 m/kt1/3 DOB. Defining “contact” is an issue; for example, a bomb’s “center of energy” does not fully describe the orientation of a staged device. The size of a hot, nuclear source is also important, since the absorption of radiation does not scale with yield. DTRA has adopted 0.05 m/ktl/3 as a standard value for the HOB of a contact (nose-down) burst to avoid elaborate numerical simulations of the energy-coupling process for each weapon type and geology.
-
Site geology and damage criterion. The type of geologic media in which the source energy is deposited (dry/wet soil/rock) affects coupled energy. Reflections and/or rarefactions occur as the ground shock propagates and impinges on stiffer or softer geologic layers. Layering can significantly influence the range to effect for a particular criterion of damage (i.e., peak overstress, peak particle velocity, or peak free-field strain). The influence of the lethality criterion is discussed below (see the subsection entitled “Response and Vulnerability of the Target Facility”).
To be fully contained (i.e., with no venting), a 300 kiloton weapon would need to be buried about 800 meters6,7 and the emplacement hole would need to be carefully stemmed.8 Because the practical penetration depth for an EPW is but a small fraction of the depth for full containment and the penetration hole would not be stemmed, there will be surface venting, prompt and residual nuclear radiation, and fallout effects from an EPW. For maximum energy coupling, analysts are most interested in the maximum depths. As can be seen in Table 3.1, which shows maximum empirically estimated EPW depths, none of the depths is great enough to contain an EPW nuclear burst, even if the penetration hole is stemmed. These effects are discussed later in this chapter.
There is a reasonably extensive experimental database, Effects Manual-1 (EM-1),9 covering the various physics regimes governing the energy-coupling process. Analytical tools are fairly well advanced also. Uncertainties associated with estimates of energy coupling are far greater for near-surface airbursts than for buried bursts and depend on how well the actual burst location and details of weapon energy output are known. For present purposes with the bomb design fully prescribed and a DOB of ≥3 meters assumed, the effective ground-shock-producing yield can be reliably estimated to within about 20 percent. The greatest uncertainties in yield equivalency are in the immediate vicinity of the ground surface, where coupling is ill-defined at the actual air-ground interface (shown in the region ±0.05 m/kt1/3 in Figure 4.1). Uncertainties exceed a factor of 2 for bursts of small heights where, of course, the ground-coupled energy is considerably less.
Shock Propagation Through the Ground to the Target Facility
Shock propagation through the ground to the target facility depends on weapon yield and coupled energy, stratigraphy of the target site, and properties of the intervening geologic materials, including joints and fault patterns. The ground-shock environment transmitted to the target facility is described in terms of material stresses, strains, particle velocities, and displacements (time-dependent details and peak values), as well as discontinuous (block) motions that can occur along joint and fault surfaces.
DTRA and the Department of Energy (DOE) weapon laboratories have invested considerable resources over the years to develop computational methods for predicting the ground-shock environments at depth from both high-explosive and nuclear bursts. The problem is complicated owing to various shock attenuation mechanisms, such as inelastic effects, hysteresis, and fracture and dilatation, and to geometric effects due to divergence, layering, interfaces, faults, and joints. Substantial progress has been made in the modeling of these processes, and there have been a number of successful predictions of large-scale, high-explosive events as well as underground (cavity) nuclear events. However, these
correlations were obtained for tests performed in media that were easily accessible for extensive pretest measurement of properties. This will rarely, if ever, be the case in targeting applications for which limited site geologic descriptions are only imprecisely known at best. Moreover, significant changes in geologic properties can occur locally as well. Thus, estimates of peak velocity or stress at depth should not be expected to be accurate to better than a factor of 2.
Response and Vulnerability of the Target Facility
Assessment of the response and vulnerability of the target facility introduces still additional uncertainty beyond that already discussed. Generally speaking, the strength of the target depends on the type, physical properties, and quality (jointing) of the surrounding geology; the method of construction and shock orientation; and the design of any internal liners, including those for possible shock isolation of internal components. The tunnel appears to be stronger under “end-on” loading (when the shock strikes at a glancing angle to the tunnel’s longitudinal axis) than when the tunnel is struck side-on.
All of the schemes for predicting tunnel damage are underpinned by a limited applicable experimental database of eight underground nuclear tests (UGTs) in which tunnels of various construction types were exposed to damaging ground-shock levels in a few types of rock geologies. The liner construction illustrated in the lower right of Figure 4.2 refers to various levels of protection, the least being reinforcement of the bare tunnel walls with rock bolts and wire mesh (an extension of what typically is done for safety considerations), and the greatest being either a ductile internal liner (composite lining) or an internal liner back-packed with a crushable material. Typical hardness ranges are indicated below each of the tunnel cross-sectional diagrams. Only two of these tests, Hard Hat (1962) and Pile Driver (1966), were dedicated experiments on structures in competent granite geology. The others were add-on experiments to UGTs conducted for different purposes in relatively soft tuff geology.
A summary of this experiment database is shown in Figure 4.2, which lists the UGT events and the number and types of structures tested. These data are limited to relatively small, lined and unlined tunnel sections, in either jointed hard rock (granite) or unjointed soft rock (tuff). All of the Hard Hat structures were 2 meters in interior diameter, while the Pile Driver structures ranged from 1 to 6 meters in interior diameter. The diameter of the tunnel excavation prior to construction of the liners ranged from 2 to 15 meters in both tests. In the major soft-rock tests (Mighty Epic, 1976, and Diablo Hawk, 1978) all lined tunnel and capsule structures were 1 meter in interior diameter. Typical excavated diameters were 2 meters. Also tested were 2-meter-interior-diameter X- and T-intersections connecting to 1-meter-interior-diameter tunnel stubs.
All of the experimental tunnels experienced severe damage at peak stress levels of about 1 to 1.5 kilobars or less, with the exception of some extremely hardened tunnels in granite that survived stress levels of 3 to 4 kilobars. More specifically, in the hard-rock tests, all structures in excavations of 5 meters or greater completely collapsed. A spectrum of damage occurred for structures in smaller-diameter excavations. It should be noted that the objectives of the hard-rock experiments were to develop survivable designs, and thus a number of the construction techniques investigated were of a more heroic design than would be likely for current targets. Thus, the conservatism inherent in the test designs and, to an extent, in the interpretation of results is not conservative from the perspective of vulnerability assessment. Nonetheless, the design and vulnerability assessment methods derived from this test experience underlie many of today’s target-planning procedures (e.g., determination of the ground vulnerability number (GVN) or the characterization of the hardness of underground facilities by the Defense Intelligence Agency).10
The DTRA contractor community and the DOE weapons laboratories have advanced analytical
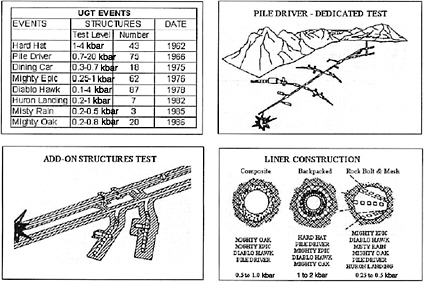
FIGURE 4.2 Database for Underground Nuclear Test (UGT) Survivability/Vulnerability (U). SOURCE: Reprinted with permission from Defense Science Board, Report on Underground Facilities, June 1998.
capabilities for assessing the vulnerability of hardened tunnels. All are calibrated in one manner or another to the applicable experimental database, as noted earlier. The use of large-scale, finite-element structural dynamic codes is commonplace. Damage typically is associated with the degree of tunnel closure (i.e., maximum circumferential strains in the tunnel liner) calibrated to the Hard Hat/Pile Driver test data. Currently, DTRA is developing an improved GVN methodology that distinguishes between crushing of the tunnel wall (and liner), a so-called global damage mode, and more local rock spall, referred to as a local damage mode. This latter damage mode is controlled by the normal component of velocity at the rock surface and is calibrated against tests of relatively shallow tunnels loaded by high-explosive charges. It is mostly applicable to unlined or minimally lined tunnels.
As part of DTRA’s program for improving GVN methodology, the global damage mode has been recalibrated to virtually the entire underground nuclear test database (i.e., EM-1) in terms of peak free-field strain in the surrounding rock, rather than peak stress, as was done in the older GVN methodology. This more physically attractive approach is made possible by modem computational capabilities. An ongoing DTRA experimental program (the Target Tunnel Defeat Advanced Concept Technology Demonstration) is intended to verify the improved vulnerability assessment method by means of a series of tunnel experiments at various scales in jointed limestone geology, the final test being of a prototype tunnel configuration loaded by a high-explosive simulation of a low-yield EPW. This test is scheduled for late 2005.
Ground-Shock Attenuation with Depth
The effectiveness of nuclear weapons against deeply buried targets can be estimated by calculating the intensity of the ground shock in the vicinity of the buried target in relation to target hardness.
Ground-shock calculations using DTRA’s state-of-the-art, two-dimensional, physics-based ground-shock code, WinGS, were done for a 5.6 megaton contact burst and a 300 kiloton EPW at 3 meters’ depth of burst in a representative homogenous granite target site; the ratio in yields was determined from the energy-coupling relationship, as discussed previously. The attenuation of peak free-field strain, stress, and velocity with depth below the ground surface resulting from these calculations is shown in Figures 4.3(a), (b), and (c), respectively. Peak stress contours for the two weapon types are compared in Figure 4.4. The general agreement between the results for the two weapon types (EPW and contact burst) supports the equivalence based on energy coupling.
Range to Effect
The hardness of tunnel-type target facilities of interest is expressed in terms of peak free-field strain (global damage mode) or peak free-field velocity (local damage mode). For an unlined or modestly lined tunnel (e.g., rock bolts and reinforced concrete liners) of 10 to 20 meters in diameter in the representative granite site, a 50 percent probability of severe damage (i.e., tunnel collapse) occurs at peak strains of 0.15 to 0.20 percent and velocities of 5 to 15 meters per second. As indicated in Figures 4.3(a) and (b), the range to effect for these hardness criteria is about 400 meters. At this range, target hardness expressed in terms of peak free-field stress is about 1 kilobar. Thus, either the 5.6 megaton contact burst or 300 kiloton EPW at 3 meters’ depth of burst can drive damaging levels of ground shock to depths of around 350 to 400 meters (range to effect) in a competent granite site. These estimates do not take into account weapon delivery accuracy (circular error probable [CEP]). As discussed later in this chapter, the probability of severe damage at these depths is about 0.5 for a 10 meter CEP. For higher probabilities of damage, say 0.95, the range to effect is reduced to around 250 meters for a 10 meter CEP, or 150 meters for a 100 meter CEP (see Figure 4.6 in the following section).
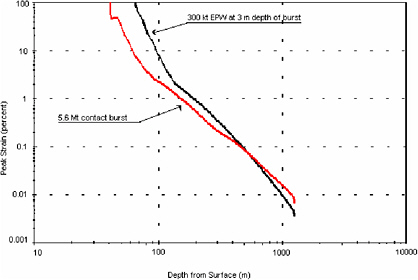
FIGURE 4.3(a) Peak strain versus target depth for 300 kt earth-penetrator weapon (EPW) and “damage equivalent” 5.6 Mt contact burst.
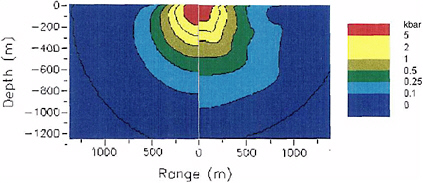
FIGURE 4.4 Peak stress contours for 300 kt earth-penetrator weapon (EPW) at 3 meters’ depth of burst (left) and “damage equivalent” 5.6 Mt contact burst (right).
Uncertainty in range to effect and associated yield factors also arises from the damage (lethality) criterion selected. This is illustrated in Table 4.1, in which range to effect is shown as corresponding to various levels of strain, stress, and velocity for the 300 kiloton EPW at 3 meters’ depth of burst and contact bursts of different yields. The upper section of Table 4.1 lists the range to effect (i.e., depth below the source) for different damage criteria and weapons. The columns labeled “Stress,” “Strain,” and “Velocity” list the down-axis range for those ground-shock properties associated with severe damage to a point target of the indicated hardness in a generic granitic rock geology for each of the listed weapons. Thus, for example, Table 4.1 indicates that a 1 kbar hard point target will be severely damaged at depths of 389 meters or less by a 300 kt EPW. If, however, the hardness of the target is only 0.25 kbar, then severe damage will occur at depths down to 501 m. The relative effectiveness of the other weapons is indicated by the other entries in the upper section of Table 4.1.
The information on range to effect for the 300 kt EPW and 5.6 Mt contact burst is obtained from Figure 4.3(b). As noted earlier, the near congruence of the two curves in Figure 4.3(b) indicates the equivalence of these weapons from a target damage perspective.
Similar information is provided in the columns of Table 4.1 labeled “Strain” and “Velocity.” DTRA’s improved assessment method characterizes the vulnerability of tunnel-like targets in terms of free-field strain or velocity normal to the tunnel surface rather than peak stress. The choice of damage criterion depends on target and weapon characteristics and is of no particular importance for the committee’s considerations here. It notes, however, that a damage criterion of 0.2 percent strain is approximately equivalent to a peak stress criterion of 1 kbar, or a peak velocity criterion of about 10 m/s. The range of effect data in Table 4.1 for strain and velocity are obtained from Figures 4.3(a) (strain) and 4.3(c) (velocity).
The lower portion of Table 4.1, “Effectiveness Ratio,” compares the relative effectiveness of the weapons listed in the upper portion of the table.
The effectiveness of one weapon in relation to another is indicated by the ratio of the range to effect for the two weapons. The yield factor based on hydrodynamic scaling is the cube of this ratio. As indicated in Table 4.1, the effectiveness of weapons with two target-damage potential equivalent (a 300 kiloton EPW at 3 meters’ depth of burst and a 5.6 megaton contact burst) can differ by about 20 percent (yield factor variation of 60 percent), depending on the damage criterion selected.
TABLE 4.1 Range to Effect, R (in meters), and Its Correspondence to Stress, Strain, and Velocity for Different Weapons
Yield/Source Location |
Damage Criterion |
|||||||
Stress |
Strain |
Velocity |
||||||
0.25 kbar |
0.75 kbar |
1 kbar |
0.15% |
0.2% |
5 m/s |
10 m/s |
15 m/s |
|
Range (meters) to effect for |
||||||||
300 kt EPWa |
501 |
435 |
389 |
411 |
370 |
464 |
359 |
307 |
5.6 Mt contact |
528 |
435 |
363 |
401 |
335 |
477 |
318 |
263 |
300 kt contact |
182 |
150 |
125 |
138 |
118 |
164 |
111 |
94 |
1 Mt contact |
275 |
226 |
186 |
208 |
175 |
247 |
166 |
140 |
|
Effectiveness Ratio |
|||||||
|
1.02 |
1.08 |
1.15 |
1.32 |
1.20 |
1.05 |
1.22 |
1.25 |
Yield factor |
1.06 |
1.26 |
1.54 |
2.29 |
1.73 |
1.15 |
1.80 |
1.97 |
|
2.75 |
2.90 |
3.11 |
2.88 |
3.14 |
2.82 |
3.23 |
3.27 |
Yield factor |
21 |
24 |
30 |
24 |
31 |
22 |
34 |
35 |
|
1.82 |
1.92 |
2.09 |
1.98 |
2.11 |
1.88 |
2.16 |
2.19 |
Yield factor |
6 |
7 |
9 |
8 |
9 |
7 |
10 |
11 |
aEarth-penetrator weapons at 3 meters’ depth of burial. |
TARGET DAMAGE PROBABILITY ESTIMATES
This section explores the destructive capabilities of various nuclear weapons—ones that are “contact burst” at the ground surface, ones that penetrate the surface, and ones that are burst in the air. The calculations were done using PDCALC (see Attachment 4.1 in this chapter), a tool used by DTRA and the United States Strategic Command (USSTRATCOM) to calculate the probability of severe target damage from nuclear weapons.
The HDBTs that are considered are those depicted in Figure 2.1. Also included are hard, surface point targets, such as missile silos. In Figures 4.5 through 4.7, the shaded areas delineate the range of target depth or target hardness of the vast majority of the 10,000 facilities that the Defense Intelligence Agency (DIA) believes to be targets, as discussed in Chapter 2. The vulnerability of deep underground targets is expressed in terms of DIA’s GVNs (or, equivalently, peak stress). For surface and near-surface targets, hardness is expressed in terms of DIA’s vulnerability numbers (VN or equivalently peak surface overpressure).
The deep underground C3 complexes and missile tunnels are between 100 meters and 400 meters deep, with the majority less than 250 meters deep. A few are as deep as 500 meters or even 700 meters in competent granitic or limestone rock.
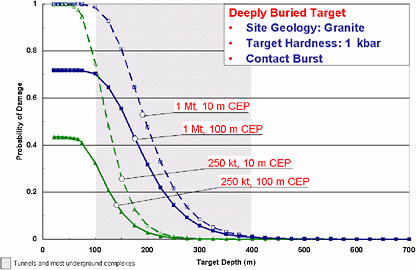
FIGURE 4.5 Effectiveness of contact bursts against some deeply buried targets. Note: CEP = circular error probable (i.e., accuracy).
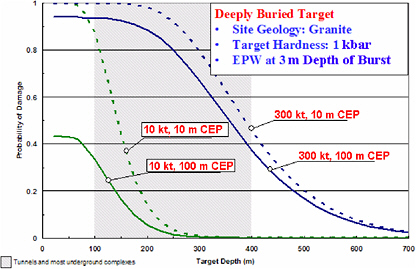
FIGURE 4.6 Earth-penetrator weapon (EPW) needs to be of sufficient yield to be effective against targets of interest. Note: CEP = circular error probable (i.e., accuracy).
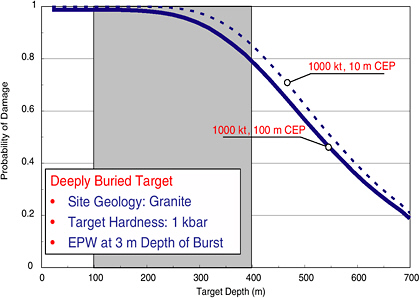
FIGURE 4.7 Earth-penetrator weapon (EPW) needs to be of sufficient yield to be effective against targets of interest. Note: CEP = circular error probable (i.e., accuracy).
Figures 4.5 through 4.7 are plots of the probability of (severe) damage of deeply buried targets in granite, as a function of the target depth. (For purposes of the calculations the targets are considered as points.) Figure 4.5 compares a 250 kiloton and a 1 megaton weapon, both of which are contact burst on the surface, for 100 meter and 10 meter CEP. Figures 4.6 and 4.7 are similar, but for 10 kiloton, 300 kiloton, and 1 megaton earth-penetrator weapons at 3 meters’ depth of burst. Examining these figures, one observes the following:
-
The effectiveness of a 250 kiloton contact burst is about the same as that of a 10 kiloton EPW, as expected from the analysis earlier in the chapter showing the 15 to 25 yield factor for equivalent ground shock.
-
Accuracy (i.e., CEP) is a critical parameter, for contact weapons, and at low yields for penetrating weapons.
-
For the target depths of interest, the most effective options examined are the 300 kiloton EPW and the 1 megaton EPW.
Included in Attachment 4.1 are additional figures and associated discussion on the influence of target hardness and CEP as well as information on nonsurface bursts.
Summary of Target Destruction
Following is a concise summary of target destruction:
-
For deeply buried targets:
-
An EPW is more effective than a contact burst of the same yield. The probability of damage for a 300 kiloton EPW at 3 meters’ DOB is equivalent to that for a 5 to 6 megaton surface-burst of the same accuracy.
-
For an EPW, yields in the range of several hundreds of kilotons to a megaton are needed to effectively hold deeply buried targets of interest at risk with a high probability of destruction.
-
-
For surface and near-surface targets of interest, as shown in Figures 4.17 through 4.19 (in Attachment 4.2), detonating a weapon at its fallout-free height of burst could effectively destroy a target without producing local fallout, although significant casualties would result from the other weapon effects (as at Nagasaki and Hiroshima).
ATTACHMENT 4.1: PROBABILITY OF DAMAGE CALCULATOR
The Probability of Damage Calculator (PDCALC) calculates the probability of damage (PD) to targets caused by, for example, overpressure, dynamic pressure, cratering, and ground-shock coupling due to nuclear weapons effects. PDCALC can also calculate weapon radius or offset to a desired probability of damage. PDCALC handles a variety of targets, including soft urban/industrial buildings, shallow buried bunkers, bridges, silos, and deeply buried tunnels. This calculator is also used to make personnel casualty assessments.
Government agencies and contractors use PDCALC as an analytical tool for planning and studies regarding nuclear weapons effectiveness, weapon requirements, and target vulnerability and survivability. DTRA manages the development and maintenance of the calculator and sponsors the PDCALC Oversight Panel and the PDCALC Users’ Group.
PDCALC is based on two Defense Intelligence Agency publications: Physical Vulnerability Handbook—Nuclear Weapons11 and Mathematical Background and Programming Aids for the Physical Vulnerability System for Nuclear Weapons.12 DIA provides the mathematical formulations embedded within PDCALC, as well as target vulnerability information used to develop target vulnerability numbers (referred to as VN). DTRA provides nuclear weapons effects data and algorithms and structural loading and response prediction methods required to develop estimates of vulnerability. While PDCALC does not contain any nuclear weapons effects models, it utilizes VN to specify the hardness of targets to various nuclear weapons effects such as overpressure, dynamic pressure, cratering, ground shock, thermal radiation, and initial nuclear radiation.
The vulnerability of a deeply buried target is given by a 10-character ground vulnerability number (GVN). GVNs are developed by DIA using the DUG1c ground-shock model. Results of depth-to-effect as a function of contact-burst yield are fit by a GVN, which is used by PDCALC. For heights of burst (HOBs) or depths of burst (DOBs) other than a contact burst, PDCALC makes use of a coupling curve to determine the equivalent contact-burst yield. This coupling curve was recently updated to incorporate the latest knowledge of energy coupling from near-surface nuclear explosions.
Currently under development is an improved GVN methodology in which DUGlc, the one-dimensional engineering ground-shock propagation code, is replaced by WinGS, a two-dimensional physics-based ground-shock code, as well as a finite-element-based tunnel response model. (See Chapter 5 for additional details.)
ATTACHMENT 4.2: INFLUENCE OF TARGET HARDNESS AND WEAPONS ACCURACY
Figures 4.5 through 4.7 assume the target hardness to be 1 kilobar. Figures 4.8 through 4.11 examine the sensitivity of the results of varying the target hardness from 0.75 kilobar to 1.5 kilobars. These figures show that for contact and buried bursts against deeply buried point targets, achieving a high probability of damage depends more strongly on improving accuracy (i.e., CEP) than on the target hardness, for the range of target hardness and yields of interest.
The scenarios for the targets examined in this study are likely to require the use of only one, or perhaps two, nuclear weapons, each of which should have an extremely high probability of damage. As discussed in Chapter 2 and earlier in this chapter, the HDBTs of interest are likely to have air defenses and to be protectively sited. Estimation of the probability, Psd, that the weapon will survive through the defensive systems and detonate where desired (air, surface, or at depth) must consider such factors. This probability is not unity (i.e., Psd will be less than 1). Since the calculated probability of damage, PD, assumes Psd = 1, which is not realistic, the calculated probability of damage PD should be reduced by some factor of less than 1 for all cases. For an EPW, the PD should be reduced more than for a similar contact-burst or airburst case because the probability of penetration to the desired depth and the probability of successful detonation are additional factors to be considered; each of these probabilities is less than 1. Figures 4.12 and 4.13 examine how good the accuracy of a contact burst or EPW must be to achieve a very high PD against a deeply buried point target with a single weapon. (Again, Psd is assumed to be 1.) Figure 4.12 demonstrates that very good accuracy—that is CEP ≤60 meters—is needed for a 1 megaton contact burst, and that targets of at most 125 meters’ depth can be held at risk with a 0.95 PD. For an EPW, Figure 4.13 shows that a single 300 kiloton weapon eases the accuracy requirements to a CEP of 110 meters or less, with targets potentially as deep as 225 meters held at risk with a 0.95 PD.
Estimated hardness levels of the command, control, communications, and intelligence (C3I) basement bunkers (Figures 4.14 through 4.18) are generally under 30 psi, the chemical weapon/biological
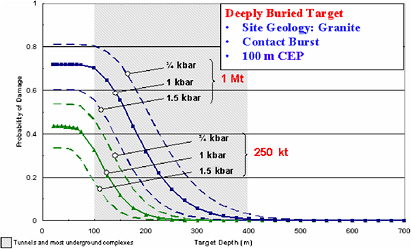
FIGURE 4.8 Contact burst with 100 meter circular error probable (CEP), or accuracy, against a deeply buried target. For a fixed CEP, effectiveness is not strongly dependent on target hardness.
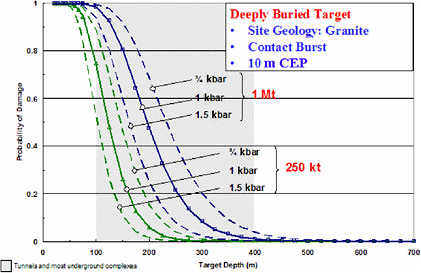
FIGURE 4.9 Contact burst with 10 meter circular error probable (CEP), or accuracy, against a deeply buried target. For a fixed CEP, effectiveness is not strongly dependent on target hardness.
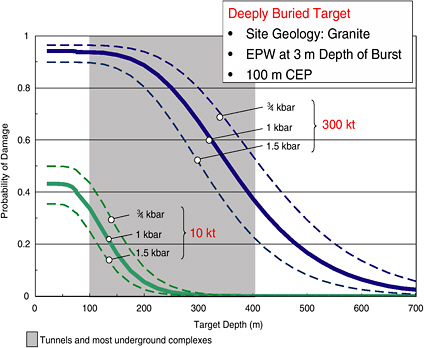
FIGURE 4.10 Earth-penetrator weapon (EPW) at 3 meters’ depth of burst with 100 meter circular error probable (CEP), or accuracy, against a deeply buried target. For a fixed CEP, effectiveness is not strongly dependent on target hardness.
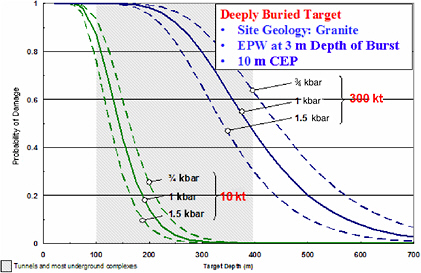
FIGURE 4.11 Earth-penetrator weapon (EPW) at 3 meters’ depth of burst with 10 meter circular error probable (CEP), or accuracy, against deeply buried target. For a fixed CEP, effectiveness is not strongly dependent on target hardness.
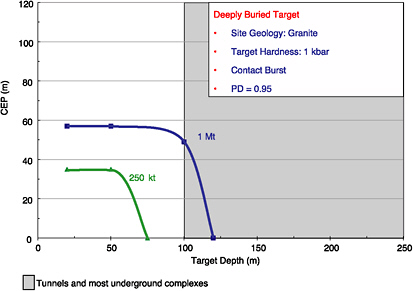
FIGURE 4.12 Contact burst against a deeply buried target. Very good accuracy and sufficient yield are required for a 0.95 probability of damage (PD) at limited target depth.
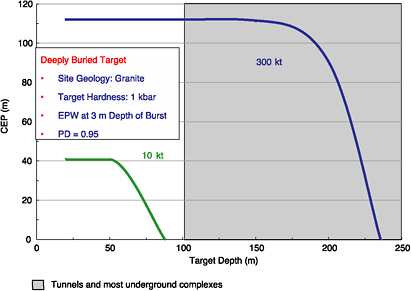
FIGURE 4.13 Earth-penetrator weapon (EPW) at 3 meters’ depth of burst against a deeply buried target. Good accuracy and sufficient yield are required for a 0.95 probability of damage (PD).
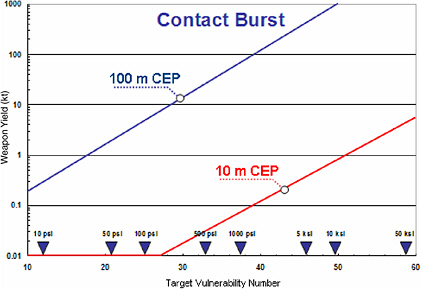
FIGURE 4.14 Contact burst against surface and near-surface point targets. Minimum yield to achieve a 0.95 probability of damage (PD) strongly depends on accuracy. Note: CEP = circular error probable; ksi = 1,000 psi.
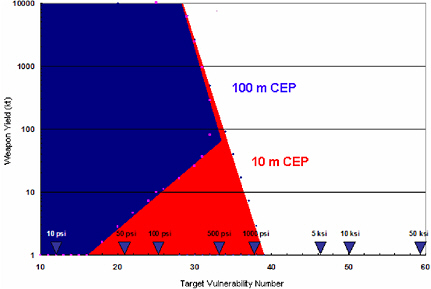
FIGURE 4.15 Fallout-free height of burst (HOB) 180 W0.4: yield to achieve a probability of damage (PD) equal to or greater than 0.95 against surface/near-surface point target. Note: CEP = circular error probable; ksi = 1,000 psi.
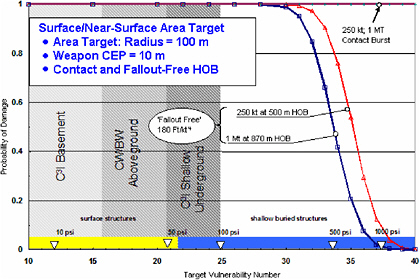
FIGURE 4.16 Large, hard, area targets vulnerable to contact or fallout-free height of burst (HOB), with weapon circular error probable (CEP) of 10 meters. Note: C3I = command, control, communications, and intelligence; CW/BW = chemical weapons/biological weapons.
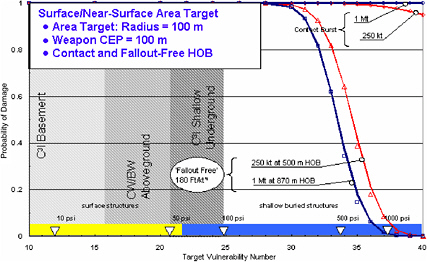
FIGURE 4.17 Large, area targets vulnerable to contact or fallout-free height of burst (HOB), with weapon circular error probable (CEP) of 100 meters.
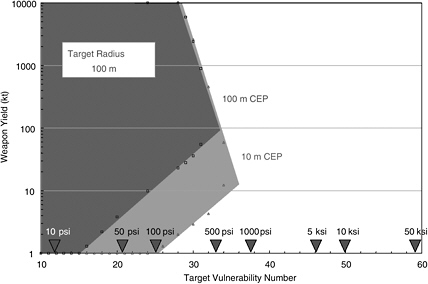
FIGURE 4.18 Fallout-free height of burst (HOB) 180 W0.4: yield for probability of damage (PD) greater than 0.95 against surface/near-surface area targets. Note: ksi = 1,000 psi.
weapon (CW/BW) aboveground bunkers are on the order of 30 to 50 psi, and the shallow underground bunkers are 50 to 100 psi. Hardness levels of missile silos are generally in the range of 1,000 psi or less.
Figures 4.14 and 4.15 focus on surface and near-surface point targets. Presumably, EPWs would not be used for such targets, especially if other options were available that were effective and could ameliorate the collateral damage due to fallout. For these, this report concentrates on surface-burst and contact-burst weapons and the so-called fallout-free height of burst. The fallout-free HOB, as its name implies, is sufficiently high that the fireball produced by the nuclear explosion does not touch the ground. It therefore is not expected to generate significantly measurable local fallout, because no surface material is entrained, lofted, or dispersed. The fall-out free HOB increases with yield, W, as W0.4.
Figure 4.14 shows that for surface and near-surface point targets, the minimum contact-burst yield needed to achieve a very high PD (i.e., 0.95) is strongly dependent on accuracy. For example, a yield of ~70 kilotons is required to achieve a PD of 0.95 against a 1,000 psi hard target if the CEP is 100 meters, whereas less than 1 kiloton is sufficient if the accuracy is 10 meters. Figure 4.15 is similar to Figure 4.14, except that the weapon is detonated at the fallout-free HOB.
Comparison of Figures 4.14 and 4.15 shows the following:
-
The minimum yield for a particular target vulnerability level is somewhat lower for the contact burst owing to higher air-blast levels predicted at the lower detonation altitude.
-
Since the lethality is proportional to W1/3, for a given PD there is a finite limit to the hardness of the target that can be destroyed at the fallout-free HOB. In this case the maximum target hardness is ~600 psi (with ~70 kilotons) for a 100 meter CEP, and ~1,000 psi (with ~1 kiloton) for a 10 meter CEP. To attack harder targets with a 0.95 PD, a higher yield detonated at a height lower than its fallout-free HOB is required.
-
The fallout-free-HOB constraint restricts the target set that can be held at risk with high probability. Further, increasing weapon yield or reducing CEP does not afford the same measurable increase in PD that may be achieved with a contact or very low airburst.
Figures 4.16 to 4.18 address hardened-surface and near-surface area targets that have a radius of ~100 meters. For these cases, an EPW is not a weapon of choice. Rather, weapons within two yields, 250 kilotons and 1 megaton, are compared at contact burst and at fallout-free HOB. Figure 4.16 assumes a 10 meter CEP, Figure 4.17 a 100 meter CEP.
The hardness levels of interest are shaded in Figures 4.16 and 4.17, with the most numerous targets in the ~5 psi to ~40 psi range. The figures show that for the target hardness range of greatest interest, any of the weapons considered will have a very high PD. The 250 kiloton weapon at its fallout-free HOB (i.e., 500 meters) is more effective than a 1 megaton weapon at its fallout-free HOB of 870 meters. This conclusion follows from cube root scaling (i.e., 250 kilotons at 500 meters gives the same peak overpressure as 1 megaton at 870 meters). Thus, the 1 megaton fallout-free HOB results in a correspondingly lower overpressure on the ground at comparable ranges. For the target hardness levels of most interest, the CEP is not especially relevant because of the extent of the target’s size. The CEP will matter more as the area of the target shrinks.
Figure 4.18 is similar to Figure 4.15, except that the surface and near-surface targets are treated as area targets for this calculation. For targets of hardness ≤100 psi, a PD of at least 0.95 can be achieved with a weapon of less than a 10 kiloton yield and no fallout if the weapon is detonated at its fallout-free HOB. Similarly, a weapon with a yield of 10 kilotons to 100 kilotons, depending on the CEP, could destroy a 500 psi target with PD ≥0.95, with no fallout.
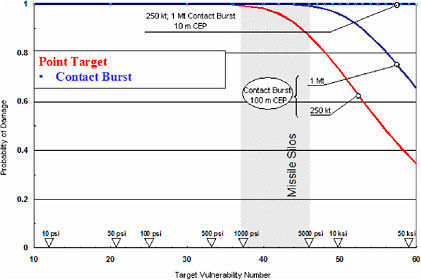
FIGURE 4.19 Accuracy and yield are important for hard point target destruction.
Figure 4.19 treats very hard point targets, such as missile silos. Only a contact burst would be effective. This figure shows the strong dependence of PD on accuracy and yield.
NOTES
1. |
Defense Nuclear Agency. 1991. Effects Manual-1 (EM-1): Capabilities of Nuclear Weapons (U), DNA-EM-1, Alexandria, Va., December (Classified). |
2. |
Ground-shock-coupled energy includes the additional energy coupled to the ground by surface air blast for height of burst detonations. |
3. |
The limiting equivalent yield factors are 99.4 and l/fgs(−0.05) = 49.9 for yield and ground-shock-coupled energy, respectively. |
4. |
Equivalence in terms of target damage criteria is discussed in the section entitled “Uncertainties in Weapon Effectiveness, Energy Coupling, and Ground-Shock Range to Effect.” |
5. |
Shel Schuster and Hal Zimmerman, 2003, “A White Paper on Energy Coupling from Nuclear and High Explosive Sources,” Titan Systems Corp., San Diego, Calif., May 20. Also discussions with Dr. Zimmerman. |
6. |
Based on a historical rule that requires a minimum depth of burst of 183 meters or a scaled depth of burst of 122 m/kt1/3 to ensure containment at the Nevada Test Site for a carefully stemmed emplacement hole. |
7. |
R.W. Terhune. 1978. Analysis of Burial Depth Criteria for Containment, UCRL 52395, Lawrence Livermore National Laboratory, Livermore, Calif., January. |
8. |
The term “stemmed” means that the emplacement hole is very carefully backfilled in an attempt to prevent the release of radioactivity via the emplacement hole. It implies that multiple cemented plugs were inserted in addition to filling the emplacement hole completely from the device location to the ground surface. |
9. |
Defense Nuclear Agency. 1991. Effects Manual-1 (EM-1), Chapter 3, “Cratering, Ejecta and Ground Shock,” DNA-EM-1-CH-3, Alexandria, Va., December. |
10. |
VN, vulnerability number, is a generic name given to a set of alpha numerics that describes the vulnerability of a target to a nuclear weapon. VNTK, a specific vulnerability number, is assigned by DIA to all facilities and personnel. DIA uses |