6
Human and Environmental Effects
EFFECTS ON HUMANS
The health effects of nuclear explosions are due primarily to air blast, thermal radiation, initial nuclear radiation, and residual nuclear radiation or fallout.
-
Blast. Nuclear explosions produce air-blast effects similar to those produced by conventional explosives. The shock wave can directly injure humans by rupturing eardrums or lungs or by hurling people at high speed, but most casualties occur because of collapsing structures and flying debris.
-
Thermal radiation. Unlike conventional explosions, a single nuclear explosion can generate an intense pulse of thermal radiation that can start fires and burn skin over large areas. In some cases, the fires ignited by the explosion can coalesce into a firestorm, preventing the escape of survivors. Though difficult to predict accurately, it is expected that thermal effects from a nuclear explosion would be the cause of significant casualties.
-
Initial radiation. Nuclear detonations release large amounts of neutron and gamma radiation. Relative to other effects, initial radiation is an important cause of casualties only for low-yield explosions (less than 10 kilotons).
-
Fallout. When a nuclear detonation occurs close to the ground surface, soil mixes with the highly radioactive fission products from the weapon. The debris is carried by the wind and falls back to Earth over a period of minutes to hours.
The first three of these effects are “prompt” effects, because the harm is inflicted immediately after the detonation. By contrast, the radiation dose from fallout is delivered over an extended period, as described in Chapter 5. Most of the dose from fallout is due to external exposure to gamma radiation from radionuclides deposited on the ground, and this is the only exposure pathway considered by the computer models that the Defense Threat Reduction Agency (DTRA) and Lawrence Livermore National Laboratory (LLNL) used to estimate health effects for this study. Below is a discussion of the possible
contribution of other exposure pathways, such as inhalation of contaminated air and consumption of contaminated water and food, to the total radiation dose received by humans.
Radiation has both acute and latent health effects. Acute effects include radiation sickness or death resulting from high doses of radiation (greater than 1 sievert [Sv], or 100 rems) delivered over a few days. The principal latent effect is cancer. Estimates of latent cancer fatalities are based largely on results of the long-term follow-up of the survivors of the atomic bombings in Japan. The results of these studies have been interpreted by the International Commission on Radiological Protection (ICRP)1 in terms of a lifetime risk coefficient of 0.05 per sievert (5 × 10−4 per rem), with no threshold.2 For the present study, acute radiation effects were estimated by both DTRA and LLNL; latent cancer deaths were estimated only by LLNL.
The computer models used by DTRA and LLNL were developed primarily to estimate effects on military personnel rather than for civilian populations. Thus, there is no consideration of the presumed greater sensitivity to radiation of the very young and the elderly. Also, there is no consideration of the sensitivity of the fetus. From the experience in Japan, it is known that substantial effects on the fetus can occur, and these effects depend on the age (stage of organogenesis) of the fetus.3 One such effect is mental retardation. The transfer of radio nuclides to the fetus resulting from their intake by the mother is another pathway of concern. Radiation dose coefficients for this pathway have been published by the ICRP.4
Another long-term health effect that is not considered here is the induction of eye cataracts. This effect has been noted in the Japanese studies and also in a study of the Chernobyl cleanup workers.5
Compared to the fatalities from prompt, acute fallout and latent cancer fatalities, the absolute number of effects on the fetus is small and is captured within the bounds of the uncertainty. The number of eye cataracts, based on the experience of the Chernobyl workers, is not small. The occurrence of eye cataracts in the now aging Japanese population is several tens of percent among those more heavily exposed.
Finally, there has been a recently confirmed finding that the Japanese survivors are experiencing a statistically significant increase in the occurrence of a number of noncancer diseases,6 including hypertension, myocardial infarction, thyroid disease, cataracts, chronic liver disease and cirrhosis, and, in females, uterine myoma. There has been a negative response in the occurrence of glaucoma. A nominal risk coefficient for the seven categories of disease is about 0.9 Sv−1 (0.009 rem−1). The largest fraction of the risk is due to thyroid disease.
Thermal Radiation from Underground Bursts
Thermal radiation may make fire a collateral effect of the use of surface burst, airburst, or shallow-penetrating nuclear weapons. The potential for fire damage depends on the nature of the burst and the surroundings. If there is a fireball, fires will be a direct result of the absorption of thermal radiation. Fires can also result as an indirect effect of the destruction caused by a blast wave, which can, for example, upset stoves and furnaces, rupture gas lines, and so on. A shallow-penetrating nuclear weapon of, say, 100 to 300 kilotons at a 3 to 5 meter depth of burst will generate a substantial fireball that will not fade as fast as the air blast.
Detonation of a nuclear weapon in a forested area virtually guarantees fire damage at ranges greater than the range of air-blast damage. If the burst is in a city environment where buildings are closely spaced, say less than 10 to 15 meters, fires will spread from burning buildings to adjacent ones. In Germany and Japan in World War II, safe separation distance ranged from about 30 to 50 feet (for a 50 percent probability of spread), but for modern urban areas this distance could be larger. This type of damage is less likely to occur in suburban areas where buildings are more widely separated.
Once started, fire spread continues until the fire runs out of fuel or until the distance to the next source of fuel is too great. Thus, fire caused directly by thermal ignitions, fire caused indirectly by disruptive blast waves, and spread of fire are all potential, but uncertain, effects.
Illustrative Example: Washington, D.C.
The area over which casualties would occur as a result of the various weapon effects outlined above depends primarily on the explosive yield of the weapon and the height or depth of the burst. The areas affected by initial nuclear radiation and fallout also depend on the design of the weapon (in particular, the fraction of the yield that is derived from fission reactions), and, in the case of fallout, on weather conditions during and after the explosion (notably wind speed and direction, atmospheric stability, precipitation, and so on), terrain, and geology in the area of the explosion. The following calculations assume that the entire population is static and in the open.
As an illustrative example,7 Figure 6.1 shows the area over which an individual in the open would face a 10, 50, and 90 percent chance of death or serious injury8 from the prompt effects of a 10-kiloton earth-penetrator weapon (EPW) detonated at a depth of 3 meters and from the prompt effects of a 250 kiloton surface burst. (As discussed in Chapter 5, both of these weapons would produce a ground shock of about 1 kilobar at a depth of 70 meters.) These contours, which were produced by the DTRA using the Hazard Prediction and Assessment Capability (HPAC) code, are shown on a map of Washington, D.C., for scale. Figure 6.2 is similar, but also includes the probability of death or serious injury from acute exposure to external gamma radiation from fallout, for illustrative weather conditions, assuming hypothetically that 50 percent of the weapon yield is derived from fission and that a static population is in the open.
Figure 6.3 compares the numbers of casualties (deaths and serious injuries) due to prompt and acute effects of fallout from the use of both weapons. Under these conditions and assumptions, the 10 kiloton EPW is estimated to result in about 100,000 casualties, compared with 800,000 casualties for the
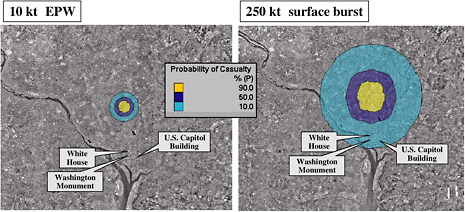
FIGURE 6.1 Illustrative example: The area over which an individual in the open would face a 10, 50, and 90 percent chance of death or serious injury from the prompt effects of a 10 kiloton earth-penetrator weapon (EPW; left) and a 250 kiloton surface burst (right) detonated at 7:00 p.m. on July 14, 2004, in Washington, D.C. SOURCE: Estimates prepared for the committee by the Defense Threat Reduction Agency.

FIGURE 6.2 Illustrative example: The area over which an individual in the open would face a 10, 50, and 90 percent chance of death or serious injury from the prompt effects of fallout from a 10 kiloton earth-penetrator weapon (EPW; left) and a 250 kiloton surface burst (right) detonated at 7:00 p.m. on July 14, 2004, in Washington, D.C. SOURCE: Estimates prepared for the committee by the Defense Threat Reduction Agency.

FIGURE 6.3 Illustrative example: Comparison of the number of casualties (deaths and serious injuries) from prompt and acute effects of fallout from a 10 kiloton earth-penetrator weapon (EPW) and a 250 kiloton surface burst detonated at 7:00 p.m. on July 14, 2004, in Washington, D.C. SOURCE: Estimates prepared for the committee by the Defense Threat Reduction Agency.
250 kiloton surface burst. Thus, in this example the use of an EPW would reduce casualties by about a factor of eight compared with a surface burst with equal destructive capacity against a buried target. Fallout is responsible for about 75 percent of the casualties from the 10 kiloton explosion compared with about 60 percent of the casualties from the 250 kiloton explosion.
The hazard to people entering the area after the explosion in these scenarios would be due largely to external gamma radiation from fallout. This hazard decreases rapidly with time: the dose rate after 1 week is 10 times less than the dose rate 1 day after the explosion, and after 2 months it is reduced by an additional factor of 10. Figures 6.4 and 6.5 illustrate this decay for the cases described above (the 10 kiloton EPW and the 250 kiloton surface burst), respectively, showing the areas exceeding a dose rate of 0.01, 0.1, 1, and 10 millisieverts per hour (1, 10, 100, and 1,000 millirems per hour) at 1 day, 1 week, 1 month, and 6 months after the explosion.
To put the dose rates referred to above in perspective, a person who remained indefinitely in an area where the dose rate was 1 millirem per hour at the time of that person’s entry into the area would receive a total dose of less than 50 millisieverts (5 rems), which is the annual dose limit for U.S. nuclear workers.9 Thus, military personnel could enter the unshaded areas shown in Figures 6.4 and 6.5 at the times indicated with minimal risk. Depending on the risk that is judged acceptable by commanders,

FIGURE 6.4 Illustrative example: Areas within which the dose rate from external gamma radiation exceeds 1, 10, 100, and 1,000 millirems per hour at 1 day, 1 week, 1 month, and 6 months after the detonation of a 10 kiloton earth-penetrator weapon at 7:00 p.m. on July 14, 2004, in Washington, D.C. SOURCE: Estimates prepared for the committee by the Defense Threat Reduction Agency.

FIGURE 6.5 Illustrative example: Areas within which the dose rate from external gamma radiation exceeds 1,10, 100, and 1,000 millirems per hour at 1 day, 1 week, 1 month, and 6 months after the detonation of a 250 kiloton surface burst at 7:00 p.m. on July 14, 2004, in Washington, D.C. SOURCE: Estimates prepared for the committee by the Defense Threat Reduction Agency.
soldiers could enter shaded areas for various periods of time. For example, a soldier entering the 10 millisieverts per hour (1,000 millirems per hour) contour 1 day after the explosion would accumulate a total dose of about 0.25 sievert (25 rems) over the next 2 days and 0.50 sievert (50 rems) over the next 2 weeks. U.S. regulatory guidelines allow doses of up to 0.25 sievert (25 rems) in lifesaving emergency situations, and the U.S. National Council on Radiation Protection and Measurements (NCRP) recommends that doses up to 0.50 sievert (50 rems) be allowed in such situations provided that individuals are aware of the risks.10 Two reports from the Institute of Medicine address the U.S. Army guidance for situations in which troops might receive as much as 0.70 sievert (70 rems ).11 Doses of 0.25 to 0.70 sievert (25 to 70 rems) are unlikely to cause serious acute effects, but they may ultimately cause death due to cancer in 1 to 3 percent of those exposed (in addition to the roughly 20 percent lifetime risk of dying of cancer from other causes).
Other Targets and Weapons Yields12
The estimates shown in Figures 6.1 through 6.5 apply only to a particular set of assumptions about target location, weather, and weapons used to attack the target. The number of civilian casualties that would result from an attack depends on many variables, including the following: the distribution of the population around the point of detonation and the degree of sheltering that they have against blast, thermal, and radiation effects; weapon yield and design; height or depth of burst; and weather conditions during and after the explosion. As shown below, the estimated number of casualties ranges over four orders of magnitude—from hundreds to over a million—depending on the combination of assumptions used.
To explore in a parametric way the range of possibilities, the committee selected three notional targets:
-
Target A: an underground command-and-control facility in a densely populated area 3 kilometers from the center of a city with a population of about 3 million;
-
Target B: an underground chemical warfare facility 60 kilometers from the nearest city and 13 kilometers from a small town; and
-
Target C: a large, underground nuclear weapons storage facility 20 kilometers from a small town.
In each case, the committee asked DTRA to estimate the mean number of casualties (deaths and serious injuries from prompt effects, and acute effects of fallout from external gamma radiation) resulting from attacks with earth-penetrating weapons with yields ranging from 1 kiloton to 1 megaton, for populations completely in the open and completely indoors. The means are averages over annual wind patterns, but they ignore precipitation. DTRA also estimated the mean number of casualties resulting from surface bursts with yields from 25 kilotons to 7.5 megatons. For selected cases, the committee asked the Lawrence Livermore National Laboratory to estimate the number of deaths from prompt effects and fallout, and to quantify the variability in acute and latent deaths from fallout owing to wind patterns.
For Figures 6.6 and 6.7 the calculations assume that the entire population is static and in the open. Figure 6.6 shows the estimated mean number of casualties resulting from attacks on Targets A, B, and C with surface-burst weapons and earth-penetrator weapons of a range of yields from 1 kt to 10 kt, with the EPW detonated at a depth of 3 meters, assuming a static population in the open. Note that for a given yield there is little or no difference between the effects of surface bursts and the EPWs.13 The curves for Target A are relatively flat (a factor-of-10 increase in yield produces a factor-of-2 increase in casualties) because the population is clustered around the target. The curves for Targets B and C are steeper (a

FIGURE 6.6 Estimated mean number of casualties (deaths and serious injuries) from attacks on notional targets A, B, and C using earth-penetrator weapons at 3 meters’ depth of burst and surface bursts, assuming a static population in the open. SOURCE: Estimates prepared for the committee by the Defense Threat Reduction Agency.
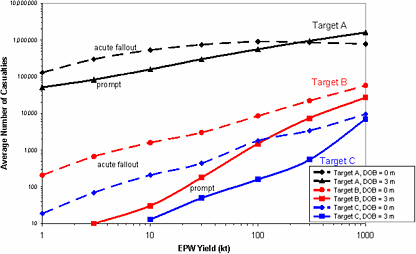
FIGURE 6.7 Estimated mean number of casualties from prompt effects and acute radiation sickness and death from fallout resulting from attacks on notional targets A, B, and C using earth-penetrator weapons (EPWs) at 3 meters’ depth of burst, assuming the entire population is in the open. SOURCE: Estimates prepared for the committee by the Defense Threat Reduction Agency.
factor-of-10 increase in yield produces a factor-of-6 to -10 increase in casualties), largely because the effects from higher-yield explosions can reach more-distant population centers.
Figure 6.7 shows the contributions of prompt effects and acute radiation sickness and death from fallout to the casualty estimates for EPWs. (The number of casualties is similar for surface bursts of the same yield.) Note that for yields of less than 300 kilotons, fallout is responsible for more casualties than are prompt effects. This is particularly true for Targets B and C, for which fallout is the only effect of low-yield explosions that can reach population centers.
It is always useful to compare model predictions against relevant experience. Fortunately, the relevant experience is very limited. In the case of the 15 kiloton device detonated over Hiroshima, an estimated 68,000 persons died and 76,000 persons were injured out of a total population of 250,000. For the 21 kiloton device detonated over Nagasaki, it is estimated that 38,000 persons died and 21,000 persons were injured out of a total population of 170,000.14 These estimates are in rough agreement with the estimated 200,000 prompt-effects casualties shown in Figure 6.7 for Target A, taking into account differences in the size of the vulnerable populations. (The Hiroshima and Nagasaki weapons were detonated at a fallout-free height of about 500 meters and therefore produced no local fallout.)
As mentioned, the results shown in Figures 6.1 through 6.7 assume that the entire population is static and in the open. Assuming that the entire population remains indoors and is thereby shielded from radiation reduces mean total casualties by a factor of up to 4 for Target A, and by a factor of 2 to 8 for Targets B and C. Not accounted for are post attack movement or evacuation of the population, but it is unlikely that individuals could, by fleeing the area of an attack, reduce their exposure to fallout significantly more than by remaining indoors. Indeed, some people might greatly increase their exposure to fallout if they were to move through highly contaminated areas, as might occur if a major road out of the city were directly under the path of the cloud. Thus, in a population that has received no warning of an attack, the actual effects of sheltering and evacuation are likely to lie between the two extremes for a population that is assumed to be entirely indoors and one that is assumed to be entirely outdoors.
The use of an EPW instead of a surface-burst weapon generally will result in fewer casualties, because the yield of the EPW can be 15 to 25 times smaller than the yield of a surface-burst weapon for a given level of damage against a hard and deeply buried target (HDBT). Figure 6.8 shows the ratio of the mean number of casualties estimated for a surface burst to the mean number estimated for an EPW with a yield 25 times smaller, for Targets A, B, and C. For Target A, casualties are reduced by a factor of 7 at low yields appropriate for target depths of less than 100 meters and by a factor of 2 at high yields and deeper targets. For Target B, casualties are reduced by a factor of 10 to 30, and for Target C, by a factor of 15 to 60, depending on the yield and assumptions about shielding. In general, the reduction factor is larger for targets in rural or remote areas.
The DTRA results presented above do not include latent cancer deaths from fallout. The committee asked LLNL to estimate the mean number of latent cancer deaths for Targets A and B, for yields from 10 to 300 kilotons.15 In the case of Target A, the inclusion of latent cancer deaths increased the total estimated number of fatalities by less than 20 percent. In the case of Target B, however, the inclusion of cancer deaths doubled the total number of fatalities. Including cancer deaths has little effect on the ratios shown in Figure 6.8.
The results given in Figures 6.6 through 6.8 are averages over annual wind patterns. Casualties from fallout can be substantially higher or lower, depending on the particular wind conditions during and immediately following the attack. Figures 6.9(a) and (b) show the variation in the number of deaths due to acute and latent effects from fallout from a 300 kiloton EPW on Targets A and B, respectively, as a function of wind direction. For Target A, estimated fatalities from fallout vary by more than an order of magnitude depending on wind direction, ranging from 90,000 to 800,000 for acute effects and from

FIGURE 6.8 Ratio of the estimated mean number of casualties from a surface burst to the mean number from an earth-penetrator weapon (EPW) with a yield 25 times smaller, for notional targets A, B, and C, assuming a static population entirely in the open or entirely indoors. SOURCE: Estimates prepared for the committee by the Defense Threat Reduction Agency.
30,000 to 200,000 for latent effects; total fatalities, however, vary by less than a factor two, from 1 million to 2 million. For Target B estimated fatalities from fallout vary by more than two orders of magnitude depending on wind direction, from 3,000 to 1 million for acute fatalities, and ranging from 3,000 to 300,000 for latent fatalities; total fatalities vary by a factor of 50, from about 15,000 to 800,000. Similarly large variations in fatalities are also possible if the target is just outside a major city. For example, if the detonation is moved 30 kilometers northwest of Target A (hereafter referred to as Target A), total fatalities vary from 50,000 to nearly 2 million, depending on whether the wind blows away from or toward the city center. Note that these estimates do not include the effects of precipitation, which would wash out and concentrate fallout in particular areas (which may or may not be populated). The committee expects that including the effects of precipitation would make the weather-related variability in the estimated number of casualties significantly greater than is suggested by this analysis. Of course, as mentioned frequently, Figure 6.9(a) and (b) are model runs and therefore are subject to the sources of uncertainty described in this report and emphasized in Chapter 8.
Figures 6.10(a) and (b) use the information in Figures 6.9(a) and (b), together with the likelihood that the wind blows in each direction, to compute the probability of exceeding a given number of deaths due to acute and latent effects from fallout, as well as from all effects, for attacks with a 300 kiloton EPW on Targets A and B. In the case of Target A, for example, the 50 percent confidence interval for deaths due to acute effects of fallout (based solely on variability in wind direction) is 130,000 to 600,000; that is, there is a 75 percent chance of exceeding 130,000 deaths from acute effects of fallout, and a 25 percent chance of more than 600,000 deaths. The 50 percent confidence interval for total fatalities is considerably narrower: 1.1 million to 1.6 million. If the detonation is moved 30 kilometers northwest of Target A, the confidence intervals are much wider: 13,000 to 700,000 for deaths from acute
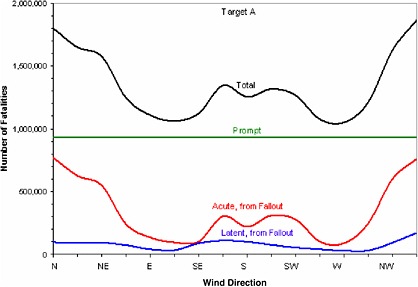
FIGURE 6.9(a) Variation in the estimated number of fatalities due to acute and latent effects from external gamma radiation from fallout from a 300 kiloton earth-penetrator weapon at 3 meters’ depth of burst on notional target A as a function of wind direction, assuming that the population is in the open. SOURCE: Estimates prepared for the committee by the Lawrence Livermore National Laboratory.

FIGURE 6.9(b) Variation in the estimated number of fatalities due to acute and latent effects from external exposure to gamma-radiation fallout from a 300 kiloton earth-penetrator weapon at 3 meters’ depth of burst on notional target B as a function of wind direction, assuming that the population is in the open. SOURCE: Estimates prepared for the committee by the Lawrence Livermore National Laboratory.
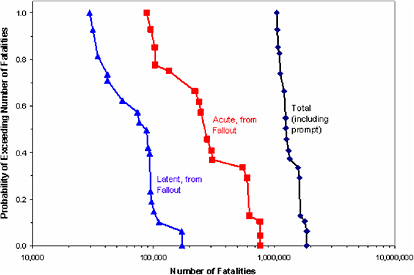
FIGURE 6.10(a) The probability of exceeding a given number of deaths due to acute and latent effects from external exposure to gamma-radiation fallout from a 300 kiloton earth-penetrator weapon at 3 meters’ depth of burst on notional target A, assuming that the population is in the open. SOURCE: Estimates prepared for the committee by the Lawrence Livermore National Laboratory.

FIGURE 6.10(b) The probability of exceeding a given number of deaths due to acute and latent effects from external exposure to gamma-radiation fallout from a 300 kiloton earth-penetrator weapon at 3 meters’ depth of burst on notional target B, assuming that the population is in the open. SOURCE: Estimates prepared for the committee by the Lawrence Livermore National Laboratory.
effects of fallout; 50,000 to 160,000 for deaths from latent effects from fallout; and 60,000 to 900,000 for total fatalities. For Target B, the corresponding intervals are 9,000 to 40,000 for deaths from acute effects of fallout; 10,000 to 60,000 for deaths from latent effects from fallout; and 20,000 to 90,000 for total fatalities.
Although the committee has not done a comprehensive analysis of the effect of wind direction for a wide range of yields, it is apparent that the casualty-reduction factor (the ratio of number of casualties for a surface burst to that for an EPW with a yield 25 times smaller) could be considerably lower or higher than the mean ratios given in Figure 6.8, depending on wind direction. For example, Figures 6.11(a) and (b) give total fatalities for a 10 kiloton EPW and a 250 kiloton surface burst for Targets A and B, respectively. Total fatalities are 10 to 40 times higher for the surface burst for Target A, depending on wind direction, with a mean 20 times higher. For Target B, the fatality ratio varies from 4 to 40, with a mean of 16; for comparison, the mean casualty ratio given in Figure 6.8 (from DTRA’s HPAC code) is 18.
The model runs show significant fatalities from both an EPW and a surface-burst weapon. The numbers are larger when the attack is near a population center and if a wind that would blow the fallout into the population center is introduced in the calculations. Figures 6.11(a) and (b) show that, for a given wind direction, the estimated number of fatalities is significantly smaller for the lower-yield EPW. It is also worth noting, however, that with unfavorable winds the lower-yield EPW would cause about as many deaths as would the higher-yield surface burst with favorable winds. For example, 40,000 deaths result from attacks on Target A from the 10 kiloton EPW with the wind blowing from the west and the 250 kiloton surface burst with the wind blowing from the east. Similarly, 15,000 deaths result from attacks on Target B from the 10 kiloton EPW with the wind blowing from the southeast and the 250 kiloton surface burst with the wind blowing from the northwest. These numbers suggest that wind direction can be as important as a 25-fold difference in yield in determining civilian casualties from attacks in which fallout is the primary health hazard.16 However, Figures 6.11(a) and (b) also show that for the same wind direction, with few exceptions, the number of fatalities from the surface burst are significantly larger than the number from the EPW. These comparisons indicate the sensitivity to wind of collateral damage to populations. However, an unfavorable wind for an EPW is, of course, also an unfavorable wind for a surface burst; the same is true for favorable winds. A population center downwind of either weapon is an unfavorable situation.
Fallout Exposure Pathways Not Considered
As noted above, the estimates produced by DTRA and LLNL of the numbers of deaths and injuries due to fallout include only the external gamma-ray dose from the deposition of fallout particles on ground surfaces.17 These estimates do not include external doses of radiation from the passing cloud or internal doses of radiation from the inhalation of contaminated air or ingestion of contaminated food or water. The contribution of these exposure pathways to the acute radiation dose usually is not substantial and would not significantly alter the estimates presented above. Under some conditions, however, the contribution of other exposure pathways to the risk of latent cancer could be significant. Here the contribution of these other exposure pathways is reviewed in a semiquantitative manner.
External Dose from the Passing Cloud
For underground, surface, or near-surface nuclear explosions, the radioactive fallout is mixed with a large mass of ejecta in the main cloud or base surge. These clouds are dense, and most of the mass at

FIGURE 6.11(a) The estimated number of total fatalities (deaths due to prompt effects, plus acute and latent effects from fallout) from attacks with a 10 kiloton earth-penetrator weapon (EPW) at 3 meters’ depth of burst and a 250 kiloton surface burst on notional target A (30 kilometers northwest of target A). SOURCE: Estimates prepared for the committee by the Lawrence Livermore National Laboratory.

FIGURE 6.11(b) The estimated number of total fatalities (deaths due to prompt effects plus acute and latent effects from fallout) from attacks with a 10 kiloton earth-penetrator weapon (EPW) at 3 meters’ depth of burst and a 250 kiloton surface burst on notional target B. SOURCE: Estimates prepared for the committee by the Lawrence Livermore National Laboratory.
close-in locations consists of large particles that deposit rapidly. Thus, most of the external dose received by persons within several kilometers of the detonation point is due to radiation from the deposited material rather than from the airborne cloud itself. Also, at close-in distances, cloud passage occurs during a rather short period of time; this is another reason that the integrated exposure from cloud passage tends to be small relative to the long-term exposure from radionuclides deposited on the ground.
During the 1950s when atmospheric nuclear testing was conducted at the Nevada Test Site (NTS), there were a number of sets of measurements of the rate of exposure before, during, and after the passage of clouds from a variety of types of nuclear tests.18 In most cases there was no measurable exposure rate that could be attributed to exposure to the cloud itself—at least not in comparison with the exposure rate derived from exposure to material on the ground. In general, the radiation dose received from the passage of the cloud itself is not a significant fraction of the dose received as a result of total external exposure.
Inhalation of Contaminated Air
In addition to external exposure, individuals may also be exposed to radiation by inhalation of fallout particles, either during the passage of the cloud or subsequently owing to resuspension of deposited particles by wind, plowing, vehicle travel, or other disturbances of the surface. Based on measured external gamma-radiation exposure rates and air concentrations observed downwind of explosions at the NTS, the whole-body inhalation dose was calculated to have ranged for most organs from 1 to 20 percent of the dose that resulted from the ingestion of contaminated food.19 However, the relative dose to the organs of the gastrointestinal tract via inhalation can be much larger, up to 80 percent of the dose from ingestion. This larger dose is due to the entrance during cloud passage of large particles into the upper respiratory tract, from which the particles are coughed up and swallowed.
The inhalation of resuspended radionuclides is a pathway of interest under only a few special circumstances—primarily with respect to the inhalation of radionuclides that do not cross biological barriers easily but can be retained over very long periods if inhaled. The most notable example of such a radionuclide is plutonium. If a nuclear device performs correctly, plutonium has not been found to be a significant source of radiation dose.
In general, inhalation is not very significant compared with other pathways of exposure. Consideration of this pathway would not significantly increase the casualty estimates presented above.
Ingestion of Contaminated Food and Water
The consumption of contaminated water has not been found to be a significant exposure pathway following nuclear tests at the NTS. Although deposition on water surfaces does occur, it has not been a significant source of exposure because dilution is rapid for persons living downwind of the NTS. The aquatic pathway was of greater concern following the Chernobyl accident, which contaminated one of the watersheds supplying water to the Kyiv Reservoir. Even in this situation, however, the consumption of contaminated water was not a substantial pathway.
Contamination of some lake systems following the Chernobyl accident in locations as far away as Sweden and Norway was more of a problem for lakes having a large surface area, shallow depth, and limited inflow and outflow. In this case the direct consumption of water was not of interest; rather, the fish in such locations were found to have elevated levels of cesium-137. It is doubtful that a similar situation would occur following a nuclear explosion, as the amounts of long-lived radionuclides created
in an explosion are much smaller than are the amounts produced in a reactor that has operated for several years.
The consumption of food contaminated by fallout from a nuclear test, however, has proven to be a major problem both at the NTS20,21 and the Semipalatinsk Polygon, a nuclear test site in the Soviet Union.22 The nature of this problem was not fully appreciated until 1963—at about the time that atmospheric testing by the United States and the former Soviet Union was ending. By far the largest concern has been associated with iodine-131, which has a half-life of 8 days. It is by the combination of several fairly unique circumstances that this radionuclide has been the major radionuclide of concern from the viewpoint of food contamination for both nuclear weapons tests and for reactor accidents.
Substantial amounts of 131I activity are created by nuclear explosions; this radionuclide is also volatile and does not condense on particles until late, at which time it becomes associated with the surfaces of fallout particles.23 Most of the total surface activity is contained on the smaller particles, so 131I is typically transported farther. The smaller particles are also preferentially retained by vegetation,24 from which they are lost with a half-retention time of about 10 days. A milk cow, if it is receiving its full quota of food from fresh pasture, will consume per day the amount of 131I that is contained on about 50 square meters,25 and it will secrete up to 1 percent of that daily intake into a liter of milk.26 Typically, a human consuming milk will concentrate 30 percent of his or her intake into the thyroid gland. The thyroid is a very small gland, weighing about 20 grams in adults and only about 2 grams in infants. Thus, iodine is preferentially retained on vegetation, which the cow efficiently samples and rapidly secretes into milk; an infant then concentrates a large fraction of that iodine in milk into an extremely small gland, thus producing a relatively large dose.
“Backyard cows” are of more concern, as such cows typically consume more pasture as opposed to stored feed, and the owners frequently drink more than an average amount of milk. Goats are also of more concern; they graze less territory, but they secrete about 10 times more of their daily intake of iodine into 1 liter of milk. For nuclear explosions outside the United States, the consumption of milk from other animals, such as sheep, horses, and camels, should be considered. The milk-transfer factors for these animals are not well known. Scientists at the National Cancer Institute are conducting a research program to determine such factors, but the results are not yet published.27 Frequently, the milk from such animals is not consumed immediately but is made into other products, thus providing some opportunity for the 131I to decay before being consumed.
For a hypothetical device (with approximately 50 percent fission fraction, i.e., 50 percent of the explosive power from fusion) that produces an integrated external dose of 1 rad, the dose to an infant’s thyroid would be about 16 rads from the consumption of milk with 131I and a few other radionuclides (132Te, 132I, 133I, and 135I). These results are scaled from published calculations made for NTS shots.28
Other radionuclides of concern in terms of contaminated foods are 89Sr, 90Sr, and 137Cs. These share the characteristics of high fission yield (the fraction of fissions that produce the radionuclide or its precursors), volatility (of the radionuclide or its precursors), and efficient secretion into milk. Other organs of concern are the digestive tract, red bone marrow, and bone surfaces.
So far, it has been assumed for this discussion that the persons and the milk animals are collocated. This is frequently not the case. Reconstruction of thyroid dose from past events has included elaborate attempts to reconstruct sources of milk or movement of milk from one region to another.29,30 If this type of predictive assessment were to be included in an analysis of effects, it would be necessary to have a database that gave the population density of humans as well as milk animals.
It is important to note that this pathway, consumption of contaminated food, can be relatively more important for fallout from nuclear explosion accidents in nonurban areas in the sense that milk animals are more likely to be located in rural areas. The problem of contaminated milk supplies following a
nuclear accident is now widely known, especially after the Chernobyl accident.31 Thus, it is frequently possible to eliminate this pathway by a variety of means (taking animals off pasture, discarding the milk, blocking the uptake of iodine by the human thyroid by feeding large quantities of stable iodine, use of cesium binders, and so on). Such elimination of this pathway would require that local inhabitants were adequately warned; that sufficient monitoring devices, iodine supplies, and distribution systems were available; and that alternate food supplies were available.
Contamination of other types of food crops would also occur. After milk, the food of most concern is fresh, leafy vegetables. Such vegetables are efficient in capturing fallout and are typically consumed fresh on a daily basis during the growing season. This practice provides an opportunity for a direct and rapid pathway to humans following deposition of fallout but, again, this pathway can be eliminated by an informed population with an adequate infrastructure. Other types of food crops typically have less ability to capture fallout or have more indirect and longer pathways to humans. The longer pathways allow for both radioactive decay and the loss of retained material from the crops. Pathways of possible concern include the consumption of meat from grazing animals, poultry, and eggs. Grain crops are not usually of concern unless they are harvested immediately after deposition of fallout.
The consumption of contaminated food is unlikely to result in any acute health effects, but it could in some circumstances increase significantly the number of latent cancers that would be expected in the affected population. An accurate estimate of the number of latent cancer fatalities from this exposure pathway would require estimating the amount of contamination in milk and various other foods, the consumption of these foods by the population, the internal dose from each radionuclide to each organ, and the use of organ-specific risk coefficients.32
Exposure to Fallout at Very Great Distances
The computer codes used for this study do not consider deposition at very great distances. If clouds are lofted to substantial heights and later encounter precipitation systems, there can be areas of enhanced deposition very far away. Such an event occurred in the area of Troy, New York, following the NTS test Simon in April 1953.33 An area of enhanced deposition also occurred in Indiana following the Trinity test in New Mexico in 1945. This deposition was eventually detected only after contaminated straw used in the packing of x-ray film was noted to have exposed the film.34
Of more recent interest were the areas of enhanced deposition that resulted from the Chernobyl accident. Contamination was sufficiently high in areas of several countries far from the accident (e.g., Sweden, Norway, and the United Kingdom) that restrictions on food use were implemented by national authorities.35 The occurrence of such areas is difficult to predict and, depending on a country’s resources, may go undetected.
“Global fallout” is a general term that describes the injection of nuclear debris into the stratosphere. Such fallout returns to Earth slowly, and with a half-time of about 1 year, most of the short-lived radionuclides would have decayed before the fallout returned to Earth. It takes a large explosion to produce such injections, on the order of hundreds of kilotons. Much of the experience with global fallout resulted from the large tests conducted by the United States and the Soviet Union from 1961 to 1963, although earlier large tests in 1952, 1954, 1956, and 1958 also produced global fallout. Concern was largely focused on 90Sr and 137Cs, each of which has a half-life of about 30 years. Under unusual circumstances, such as the large-scale subsidence of air masses or the penetration of large thunderstorms into the stratosphere, the deposition of 131I was also noted.36 The negative worldwide reaction to global fallout was intense in the early 1960s, and this was one of the more important factors that resulted in the agreement to stop atmospheric tests by the United States, the United Kingdom, and the Soviet Union.
HEALTH EFFECTS FROM ATTACKS ON FACILITIES FOR STORING AND PRODUCING NUCLEAR WEAPONS AND RADIOACTIVE MATERIAL
The health effects resulting from attacks with conventional weapons on nuclear-weapon storage facilities depend on the detailed design of the nuclear weapons being attacked. Because the design details of enemy nuclear weapons are unknown (and could not be discussed in this document in any case), the committee cannot provide quantitative estimates.
However, if the enemy nuclear weapons are “one-point safe” (i.e., there is less than 1 chance in a million that the yield will be over 4 pounds when the high explosive is initiated and detonated at a single point), then the main risk to nearby civilian populations would result from the dispersal of radioactive material. The greatest such risks would arise from weapons containing plutonium. Even in this case, however, the dispersal of plutonium from tens of weapons would be unlikely to cause deaths or acute illnesses in civilian populations. Dispersal of plutonium could, however, result in thousands of latent cancer deaths if kilogram quantities of plutonium aerosol were dispersed in densely populated areas.37 If the weapons contain no plutonium (only highly enriched uranium), then this concern would be very much reduced. If an enemy’s nuclear weapons are not one-point safe, it is possible that a conventional attack could result in a nuclear detonation. In this case, the effects on nearby civilian population would be similar to those estimated in Figure 6.7.
If the enemy’s nuclear weapons are not certifiably one-point safe, then the assessment of possible yields is much more complicated. It seems probable that even an early-stage nuclear country or group would desire some degree of safety in order to preserve both the weapon and the nuclear material for the use for which it was intended. Consequently, other techniques will likely be employed to create safe operating conditions for the weapons. For example, the weapon components can be kept in separate locations, ready to be assembled quickly for possible use (as was done with a number of U.S. weapons). There may also be mechanical safety devices in place that lead to a low probability of unintentional detonation (even if not as quantitative as the one-point safety criterion). And many possible weapons will be “partially safe”—i.e., their one-point yield will be much less than their design yield. As a result, the probability of significant nuclear yield from a conventional attack is quite low—but cannot be completely ruled out. In that case an upper limit for the effects is similar to the limits estimated in Figure 6.7. However, the most likely outcome of such an attack is dispersal of the nuclear material, the equivalent of the dirty bomb scenario discussed below.
The dispersal of radioactive materials from a non-nuclear explosion would be possible, for example, if sympathetic detonation of high explosives led to dispersal of the radioactive material either in weapons or in a facility such as a reprocessing plant. In this case, the effects would be similar to those discussed for what have been called “radiological” weapons.
The term “radiological weapon” is extremely broad and imprecise. A radiological weapon could involve a device using any of hundreds of radionuclides, in quantities ranging from harmless to lethal, in physical and chemical forms that are easy or impossible to disperse efficiently.
Calculations done by others38 indicate that the acute effects of a “dirty bomb” containing even a potent radioactive source would in most cases not extend beyond the lethal radius of the high explosive used to disperse the radioactive material. Accordingly, the committee expects that a conventional attack on a facility containing radiological weapons or radioactive materials would be unlikely to produce a substantial number of civilian deaths or acute illnesses, beyond those caused directly by the conventional attack itself. The number of latent cancer deaths that might result from a dispersal of radioactive material would depend sensitively on the type and amount of material dispersed (as well as the density of nearby civilian populations and whether these populations were evacuated from the area after the
attack). Uncertainties in the source term make quantitative estimates impossible, but the estimates given above for plutonium dispersal indicate the consequences of the dispersal of a very large mass of highly radioactive material.
ENVIRONMENTAL EFFECTS
In addition to the health effects mentioned above, a variety of environmental effects can be expected from nuclear explosions near the ground’s surface. Following the explosion of a nuclear weapon, the fallout area is intensely radioactive. However, as noted above, the rate of external exposure to gamma radiation decreases rapidly with time, and the denial of land use due to fallout is not of great concern relative to other effects of fallout. This is in marked distinction to the situation to be expected following a major reactor accident such as that at Chernobyl,39 because of the much greater releases of long-lived l37Cs. Denial of the use of water would be expected to be of even less concern, except under very unusual circumstances, because of the very rapid dilution of fallout deposited on surface waters. It is unlikely that significant contamination of groundwater would occur, except in areas immediately adjacent to an explosion of an earth-penetrator weapon. Although underground facilities could be built below the water table and kept dry by diversion and pumping, most facilities are expected to be above the water table. The groundwater in the immediate area of an underground burst would be contaminated, but the greatest release of radioactivity would be from activated materials that are spread onto the surface. A bunker facility is highly unlikely to be built in groundwater. Groundwater is likely to be in the fallout area. However, the greatest release of radioactivity would be from activated material that is spread onto the water surface. The transport of radionuclides due to the movement of groundwater will be difficult to evaluate with any useful certainty as it is a very site-specific phenomenon. U.S. experience at the Nevada Test Site indicates that the movement of radionuclides by groundwater is quite limited, although some radionuclides have been found off-site after many decades. Such effects of groundwater will be far less than the effects of blast, fire, and on-the-ground fallout.
The radiation sensitivity of all other mammals is generally about the same as that for humans. Thus, in areas where humans are killed or injured by radiation, the same lethality for animals would be expected. If large herds of farm animals were affected, poor sanitation could become a significant problem.
Plant species have a broad range of sensitivity to radiation.40 Among the more sensitive are some species of trees, particularly pine and spruce, which are roughly as sensitive as humans are. Thus, it is conceivable that forests could be killed, which in turn could result in forest fires. The demise of the pine forest near the Chernobyl plant was one notable example of this effect.41 Other vegetation types might also die as a result of contamination within the range of concern for human lethality. It is not likely that effects in excess of that indicated for pine forests would occur.
Recently, there has been a focus on evaluating the possible effects of radiation on other members of an ecological system.42 Generally, concern is limited to the possible effects on populations of species rather than on individual members of an ecosystem. It is not expected that effects other than those mentioned above would be of significance.
In the past there has been concern that large numbers of nuclear explosions might lead to large-scale disruption of the environment, including depletion of stratospheric ozone due to nitrogen oxides produced by the fireball, and changes in climate due to the soot and other aerosols released from burning cities. These concerns are relevant only with the detonation of thousands of high-yield weapons. No significant environmental disruptions would be expected to occur beyond the areas directly affected by the prompt effects from one or a few nuclear explosions and the fallout that, depending on the amount of soil entrained and the fission fraction of the weapon(s), can persist at dangerous levels for at least a year.
EFFECTS OF ATTACKS ON CHEMICAL AND BIOLOGICAL WEAPONS FACILITIES
Earth-penetrator weapons—conventional or nuclear—provide a means to defeat or destroy hardened and/or deeply buried facilities used for the production or storage of chemical and biological agents. In this context, there are three important questions:
-
To what extent can conventional or nuclear weapons destroy such facilities or the chemical and biological agents that they contain?
-
To what extent would a conventional or nuclear attack on such a facility result in the release of chemical and biological agents?
-
If chemical or biological agents are released as a result of an attack, what would be the health consequences for the nearby civilian population?
The answers to the first two questions depend critically on detailed information about the facility, including its location, construction, and layout; the type and number of agent containers and their placement within the facility; and the amount and type of agent and the form in which it is stored. This information would provide the basis for targeting, selecting weapons, and estimating how much agent might be destroyed or released. Unfortunately, detailed information of this kind is likely to be highly uncertain or unavailable for many potential targets. Existing estimates of the amount of agent that might be destroyed or dispersed in a nuclear attack are based entirely on computer models using greatly simplified assumptions. In the case of conventional attacks, experiments also have been conducted using prototype facilities, with surrogates in place of live agent. Even with all of these qualifications, certain important points can be made:
-
It is important to distinguish between the defeat or destruction of a chemical or biological weapons facility and the destruction of the chemical or biological agent contained within it. Facilities can be defeated or destroyed without destroying the agent inside. For example, a nuclear EPW could crush a storage facility under 100 meters of rock without destroying (or releasing) any agent. Similarly, conventional weapons could collapse surface or near-surface entrances to such a facility and thereby hinder or delay the use of agents by the enemy.
-
If facilities or storage areas are penetrated by a nuclear or conventional weapon, significant degradation (thereby reducing potential releases) can be effected by heat (>1,000 degrees Fahrenheit and residence time >20 to 30 seconds).
-
The thermal destruction of chemical or biological agents requires the deposition of large amounts of heat throughout the agent. Although existing conventional earth-penetrator weapons, such as the GBU-24, can penetrate and destroy shallow buried facilities, they cannot deliver enough energy to reliably and completely destroy large stockpiles of chemical or biological agent, although they may substantially degrade the agents. Non-nuclear agent-defeat weapons now under development may ultimately prove to be more effective. However, the BLU-118B thermobaric bomb, if detonated within the chamber, may be able to destroy the agents.43
-
Nuclear weapons are capable of delivering the very large amounts of heat and radiation required to destroy large stocks of chemical and biological agents. In order for this heat and radiation to be deposited throughout the agent, the nuclear weapon must be detonated in the chamber where the agent is stored. Weapons detonated several meters above, below, or to the side of storage facilities may be much less effective in destroying the agent.
-
The manner in which the agent is stored (e.g., the types of containers, location in multiple storage
-
rooms) and the proximity of the detonation of the weapon may result in significant variations in the radiation doses and thermal histories of the agent in different parts of a facility. Given the many unknowns, a conservative analysis must presume some release of the agent in a viable form if the facility is breached, regardless of the type of weapon used.
-
The amount of agent that likely would be released is extremely difficult to estimate accurately. DTRA estimates that an attack with existing conventional weapons could cause the release in respirable form of 0.1 to 5 percent of the agent inventory.44 Calculations indicate that an attack with a nuclear weapon could result in comparable releases if the weapon was detonated close to but not within a facility, but much smaller releases if the weapon was detonated in the same room as the agent.
The consequences of a release of agent can be estimated using computer codes that model the dispersion of agent and subsequent human exposures and health effects. Although these models are similar in some ways to those used to estimate the consequences of nuclear fallout, the transport of chemical and biological agents is more complicated and more uncertain. The particle size distribution of biological agents and some chemical agents may change during transport. The persistence of both chemical and biological agents depends on temperature, humidity, exposure to ultraviolet light, precipitation, and agent-surface reactions. The importance of these factors differs for each type of agent, but for most chemical and biological agents of concern, one may expect a rapid degradation in their toxicity or viability within hours to days—minutes in the case of some biological agents—following a release into the open air. In contrast, some agents, such as anthrax spores, mustard, and lewisite, may persist for many years.
Although many studies have validated and verified the fate of chemical agents during transport, few are available for biological agents, and the fate of biological agents during transport is therefore difficult to model. In addition, the dose-response relationships are uncertain for many biological agents and often are very sensitive to the age and health status of the person exposed. As with fallout, the estimated number of casualties resulting from a given release of agent can vary across a very wide range, depending on weather conditions, the density and distribution of the population, the proportion of the population that is sheltered and/or equipped with protective gear, and the availability of prompt medical care.
Though multiple experiments using biological and chemical agent surrogates have been conducted, they provide an imperfect database. Actual experience that might be used to validate models is limited to one release of biological agent (anthrax spores) at Sverdlovsk in 1979 and one release of chemical agent (sarin) in the Tokyo subway system in 1995. Media reports of the use of chemical agents by the Iraqi government against Kurdish villages do not provide sufficient information about agent concentrations or delivery method to be useful, and the case of the letters containing anthrax sent through the U.S. Postal Service in 2001 is of limited relevance to the type of situation considered here.
At the request of the committee, DTRA estimated the average number of fatalities that would result from various releases of sarin (a nerve agent) and anthrax at three locations in the Washington, D.C., area: the city center and 10 and 50 kilometers northwest of the city center. In each case, releases of 1 to 10,000 kilograms of sarin and 1 gram to 10 kilograms of weaponized dry anthrax spores were considered, corresponding to releases of 0.001 to 10 percent of an inventory of 100 tons of sarin and 100 kilograms of anthrax. The average number of fatalities from prompt and acute effects of fallout resulting from attacks with nuclear EPWs with yields of 3 and 30 kilotons were also estimated. The population was assumed to be static and entirely in the open with no protection. The results are shown in Figures 6.12(a) through 6.12(c).
The estimated mean number of fatalities resulting from a 1,000 kilogram release of sarin (1 percent of a 100 ton inventory) ranges from about 100 to 1,000 depending on the location of the release. In

FIGURE 6.12(a) Illustrative example: Estimated mean number of fatalities from releases of sarin or anthrax at city center of Washington, D.C., compared with the mean number of fatalities resulting from 3 kiloton and 30 kiloton nuclear earth-penetrator weapon (EPW) explosions at the same location. SOURCE: Estimates prepared for the committee by the Defense Threat Reduction Agency.
contrast, the mean number of fatalities from a release of 1 kilogram of dry anthrax spores (1 percent of a 100 kilogram inventory) is about 100,000 for each of the three locations. For the reasons previously discussed (i.e., the generally fragile nature of most toxic agents), the calculated number of fatalities for the release of sarin is certainly too high.
For comparison, the estimated mean number of fatalities ranges from 7,000 to 40,000 for a 3 kiloton EPW, and from 30,000 to 130,000 for a 30 kiloton EPW, depending on the location. Because the expected number of fatalities from a relatively low yield (3 kiloton) nuclear EPW exceeds that from an extremely large (10,000 kilogram) release of sarin, it is highly unlikely that a nuclear attack would result in smaller total collateral effects than those from a conventional attack against a facility for the storage or production of chemical agents. In contrast, releases of as little as 0.1 kilogram of anthrax result in a calculated number of fatalities that is comparable to that estimated for a 3 kiloton nuclear EPW.
NOTES
4. |
International Commission on Radiological Protection. 2001. “Doses to the Embryo and Fetus from Intakes of Radionuclides by the Mother,” Annals of the ICRP, ICRP Publication 88, Vol. 31 (1-3), Pergamon Press, Oxford. |
5. |
A.K. Junk, Y. Kundiev, P. Vitte, and B.V. Worgul. 1999. Ocular Radiation Risk Assessment in Populations Exposed to Environmental Radiation Contamination, Kluwer Academic Publishers, Boston, Mass. |
6. |
M. Yamada, F.L. Wong, S. Fujiwara, M. Akahoshi, and G. Suzuki. 2004. “Noncancer Disease Incidence in Atomic Bomb Survivors, 1958-1998,” Radiat. Res., Vol. 161, pp. 622-632. |
7. |
The yield options for the proposed RNEP cover a wide range, and the committee reviewed analyses by DTRA and others that covered a wide range of EPW yields. Although the hardest and deepest targets require EPW yields of 300 to 1,000 kt, other targets of interest could be destroyed with EPWs with yields of 1 to 10 kt. The committee therefore did a parameter analysis in which the EPW yield ranged from 1 to 1,000 kt. The mean number of casualties over this range of EPW yields is shown in Figures 6.6 and 6.7. |
8. |
A “serious injury” is defined as one requiring hospitalization for 60 days or more. |
9. |
This is true for entry times of less than 6 months. |
10. |
National Council on Radiation Protection and Measurement. 1993. Limitation of Exposure to Ionizing Radiation, Report No. 116, Washington, D.C. |
11. |
Institute of Medicine. 1997. An Evaluation of Radiation Exposure Guidance for Military Operations, Interim Report, National Academy Press, Washington, D.C.; Institute of Medicine. 1999. Potential Radiation Exposure in Military Operations, National Academy Press, Washington, D.C. |
12. |
The effects discussed here are only those from the nuclear weapon and its direct effects and do not include chemical and biological agents, which are discussed in the section of this chapter entitled “Effects of Attacks on Chemical and Biological Weapons Facilities.” |
13. |
1f the depth of burst is increased from 3 meters to 10 meters, total casualties increase only by about 10 percent at high yields to 20 percent at low yields, owing largely to the increase in the amount of soil excavated by the explosion. |
14. |
Samuel Glasstone and Philip J. Dolan (eds.). 1977. The Effects of Nuclear Weapons, U.S. Government Printing Office, Washington, D.C. |
15. |
Using a risk coefficient of 0.05 per sievert, the National Atmosphere Release Advisory Center estimated cancer deaths for populations receiving doses greater than 1 millisievert (100 millirems), which is roughly equal to the average annual dose due to external radiation from cosmic rays and radionuclides in soil. This threshold was used only to limit the complexity of the calculation; the committee takes no position on whether a threshold exists in the dose-response relationship. |
16. |
The National Command Authority and the deployers have opportunities and the responsibility to execute an attack on HDBTs in ways to minimize collateral damage by taking into account wind direction as well as yield. |
17. |
Lethal beta skin burns, the major cause of fatality from acute effects of fallout at Chernobyl, are not considered. KDFOC does not consider beta burns in its analyses because burns are not considered a first-order lethality effect, like prompt and local fallout. For residual effects, it considers only whole-body gamma groundshine from fallout particles greater than 5 microns. Beta burns from such fallout particles would not be acutely lethal except in areas where gamma radiation would already have been lethal, thus, double-counting. HPAC does not include beta-induced injuries—all casualties are derived from effects of gamma radiation. The main problem with beta injuries is that the material must come into contact with skin, and HPAC has no means to determine the orientation and skin exposure posture of the population, nor the secondary beta burns received by people touching a surface contaminated with beta particles. Secondary beta burns are potentially a problem, but there is no way to determine casualties because the total population is not affected. |
18. |
H.G. Hicks. 1990. “Additional Calculations of Radionuclide Production Following Nuclear Explosions and Pu Isotopic Ratios for Nevada Test Site Events,” Health Phys., Vol. 59, pp. 515-523. |
19. |
Y.C. Ng, L.R. Anspaugh, and R.T. Cederwall. 1990. “ORERP Internal Dose Estimates for Individuals,” Health Phys., Vol. 59, pp. 693-713. |
20. |
Y.C. Ng, L.R. Anspaugh, and R.T. Cederwall. 1990. “ORERP Internal Dose Estimates for Individuals,” Health Phys., Vol. 59, pp. 693-713. |
21. |
F.W. Whicker and T.B. Kirchner. 1987. “PATHWAY: A Dynamic Food-Chain Model to Predict Radionuclide Ingestion After Fallout Deposition, Health Phys., Vol. 52, pp. 717-737; F.W. Whicker, T.B. Kirchner, L.R. Anspaugh, and Y.C. Ng. 1996. “Ingestion of Nevada Test Site Fallout: Internal Dose Estimates,” Health Phys., Vol. 71, pp. 477-486. |
22. |
K. Gordeev, I. Vasilenko, A. Lebedev, A. Bouville, N. Luckyanov, S.L. Simon, Y. Stepanov, S. Shinkarev, and L. Anspaugh. 2002. “Fallout from Nuclear Tests: Dosimetry in Kazakhstan,” Radial. Environ. Biophys., Vol. 41, pp. 61-67. |
23. |
H.G. Hicks. 1982. “Calculation of the Concentration of Any Radionuclide Deposited on the Ground by Off-site Fallout from a Nuclear Detonation, Health Phys., Vol. 42, pp. 585-600. |
24. |
L.R. Anspaugh, S.L. Simon, K.I. Gordeev, I.A. Likhtarev, R.M. Maxwell, and S.M. Shinkarev. 2002. “Movement of Radionuclides in Terrestrial Ecosystems by Physical Processes,” Health Phys., Vol. 82, pp. 669-679. |
25. |
J.J. Koranda. 1965. Agricultural Factors Affecting the Daily Intake of Fresh Fallout by Dairy Cows, UCRL 12479, Lawrence Livermore National Laboratory, Livermore, Calif. |
26. |
National Cancer Institute. 1997. Estimated Exposures and Thyroid Doses Received by the American People from Iodine-131 Following Nevada Atmospheric Nuclear Bomb Tests: A Report from the National Cancer Institute, U.S. Department of Health and Human Services, Washington, D.C. |
27. |
Steven L. Simon, National Cancer Institute, Bethesda, Md., personal communication, 2003. |
28. |
Y.C. Ng, L.R. Anspaugh, and R.T. Cederwall. 1990. “ORERP Internal Dose Estimates for Individuals,” Health Phys., Vol. 59, pp. 693-713. |
29. |
R.A. Kerber, J.E. Till, S. Simon, J.L. Lyon, D.C. Thomas, S. Preston-Martin, M.L. Rallison, R.D. Lloyd, and W. Stevens. 1993. “A Cohort Study of Thyroid Disease in Relation to Fallout from Nuclear Weapons Testing,” JAMA, Vol. 270, pp. 2076-2082. |
30. |
National Cancer Institute. 1997. Estimated Exposures and Thyroid Doses Received by the American People from lodine-131 Following Nevada Atmospheric Nuclear Bomb Tests: A Report from the National Cancer Institute, U.S. Department of Health and Human Services, Washington, D.C. |
31. |
United Nations Scientific Committee on the Effects of Atomic Radiation (UNSCEAR), 1988, Sources, Effects and Risks of Ionizing Radiation, UNSCEAR 1988 report to the General Assembly, with annexes, United Nations, New York, Sales No. E.88.IX.7; also, United Nations Scientific Committee on the Effects of Atomic Radiation (UNSCEAR), 2000, Sources, Effects and Risks of Ionizing Radiation, UNSCEAR 2000 report to the General Assembly, with scientific annexes, United Nations, New York, Sales No. E.00.IX.4. |
32. |
Such values are given in J.S. Puskin and C.B. Nelson, 1995, “Estimates of Radiogenic Cancer Risks,” Health Phys., Vol. 69, pp. 93-101. |
33. |
H.M. Clark. 1954. “The Occurrence of an Unusually High-Level Radioactive Rainout in the Area of Troy, N.Y.,” Science, Vol. 119, pp. 619-622. |
34. |
J.H. Webb. 1949. “The Fogging of Photographic Film by Radioactive Contaminants in Cardboard Packaging Materials,” Phys. Rev., Vol. 76, pp. 375-380. |
35. |
United Nations Scientific Committee on the Effects of Atomic Radiation (UNSCEAR), 1988, Sources, Effects and Risks of Ionizing Radiation, UNSCEAR 1988 report to the General Assembly, with annexes, United Nations, New York, Sales No. E.88.IX.7; also United Nations Scientific Committee on the Effects of Atomic Radiation (UNSCEAR), 2000, Sources, Effects and Risks of Ionizing Radiation, UNSCEAR 2000 report to the General Assembly, with scientific annexes, United Nations, New York, Sales No. E.00.IX.4. |
36. |
L. Machta. 1963. “Meteorological Processes in the Transport of Weapon Radioiodine,” Health Phys., Vol. 9, pp. 1123-1132. |
37. |
Steven A. Fetter and Frank von Hippel. 1990. “The Hazard from Plutonium Dispersal by Nuclear-Warhead Accidents,” Science and Global Security, Vol. 2, pp. 21-41. |
38. |
For example, see National Research Council, 2002, Making the Nation Safer, National Academies Press, Washington, D.C., p. 49. |
39. |
United Nations Scientific Committee on the Effects of Atomic Radiation (UNSCEAR). 2000. Sources, Effects and Risks of Ionizing Radiation, UNSCEAR 2000 report to the General Assembly, with scientific annexes, United Nations, New York, Sales No. E.00.IX.4. |
40. |
S. Ichikawa and A.H. Sparrow. 1967. Radiat. Bot., Vol. 7, pp. 429-441. |
41. |
United Nations Scientific Committee on the Effects of Atomic Radiation (UNSCEAR). 1996. Sources and Effects of Ionizing Radiation, UNSCEAR 1996 report to the General Assembly, with annex, United Nations, New York, Sales No. E.96.IX.3. |
42. |
United Nations Scientific Committee on the Effects of Atomic Radiation (UNSCEAR). 1996. Sources and Effects of lionizing Radiation, UNSCEAR 1996 report to the General Assembly, with annex, United Nations, New York, Sales No. E.96.IX.3. |
43. |
For additional information, see the section titled “Background” in Chapter 1. |
44. |
Todd Hann, Defense Threat Reduction Agency, personal communication, August 13, 2004. |