1
Introduction
GENESIS OF THE STUDY
Energy is essential to the functioning of society. From coal for electricity production to oil products for transportation to natural gas for space heating, every aspect of modern life depends on energy. Yet, as beneficial as energy is, its production, distribution, and consumption also have negative impacts especially on human health and the environment.
Most effects of energy are negative, but that does not imply that energy use has an overall negative impact on society. Quite the contrary; the benefits to society of U.S. energy systems are enormous. However, it was not the committee’s task to estimate those benefits that are considered largely to be “internal” because they are reflected in energy prices or government policies.
The purpose of this study was to define and evaluate external effects of energy production, use, and consumption, which refer to costs and benefits not taken into account in making decisions (such as the siting of a power plant) or not reflected in market prices (for example, the price of gasoline at the pump). Under such conditions, the actions that follow might be sub-optimal—in the sense that the full social costs of the actions are not recognized—resulting in a loss of social welfare. When market failures like these occur, there is a case for government intervention in the form of regulation, taxes, fees, tradable permits, or other instruments that will cause economic agents to recognize the external effects in their decision making.
Before such public policies are pursued, the external effects of energy
and their monetary values should be known. Thus, Congress directed the U.S. Department of the Treasury in the Energy Policy Act of 2005 (P.L. 109-58), Section 1352, to commission a study by the National Academy of Sciences that would “define and evaluate the health, environmental, security, and infrastructure external costs and benefits associated with the production and consumption of energy that are not or may not be fully incorporated into the market price of such energy, or into the Federal revenue measures related to that production or consumption.” Funding for the study was later provided through the Consolidated Appropriations Act of 2008 (P.L. 110-161).
STATEMENT OF TASK
In response to this mandate from Congress and the request from the Department of the Treasury, the National Research Council (NRC) established the Committee on Health, Environmental, and Other External Costs and Benefits of Energy Production and Consumption (see Appendix A). The Statement of Task (Box 1-1) was developed and served as the point of departure and guide for the committee’s work. In the remainder of this chapter, we define key terms in the Statement of Task and explain the general procedures followed in executing the task.
This study is one of many related to energy that the NRC has recently undertaken. In the next section, we briefly discuss those NRC studies that have informed our work, especially the America’s Energy Future (AEF) initiative, which is identified in the Statement of Task. We also briefly review previous studies on the external costs of energy.
Also in this chapter, we provide the definition of an externality—the focus and core concept of this study—and provide some examples.
The Statement of Task directed us to evaluate the externalities “associated with the production, distribution and consumption of energy from various selected sources.” We explain how we selected the sources and the particular elements of the energy system on which we focused.
The approach that we took for identifying, quantifying, and evaluating externalities “in economic terms” is explained. A discussion of “appropriate metrics from each externality category” is included.
Although the committee was not asked to “recommend specific strategies for correcting observable externalities, because those choices will entail policy judgments”—a position with which we agree—it is important to understand and to keep in mind the policy contexts in which our results may be used.
The Statement of Task anticipated some of the methodological challenges of evaluating externalities. We discuss the particular difficulties of
BOX 1-1 Statement of Task An NRC committee will define and evaluate key external costs and benefits— health, environmental, security, and infrastructure—associated with the production, distribution, and consumption of energy from various selected sources that are not or may not be fully incorporated into the market price of such energy, or into the federal tax or fee or other applicable revenue measures related to such production, distribution, or consumption. Although the committee will carry out its task from a U.S. perspective, it will consider broader geographic implications of externalities when warranted and feasible. The committee will not recommend specific strategies for internalizing observable externalities, because those choices would entail policy judgments that transcend scientific and technological considerations. In carrying out its task, the committee will include the following activities: Seek to build upon the results of the NRC initiative America’s Energy Future: Technology, Opportunities, Risks, and Tradeoffs. Identify key externalities to be assessed in the categories of human health, environment, security (including quality, abundance, and reliability of energy sources), and infrastructure (such as transportation and waste disposal systems not sufficiently taken into account by producers or consumers). Consider externalities associated with producing, distributing, and consuming energy imported from foreign sources. Define appropriate metrics for each externality category considered. Identify state-of-the-science approaches for assessing external effects (actual or expected) and expressing their effects in economic terms. Develop an approach for estimating externalities related to greenhouse gas emissions and climate change. Estimate externalities related to those changes. Present qualitative and, to the extent practicable, quantitative estimates of externalities and associated uncertainties within a consistent framework that makes the discussion of externalities and uncertainties associated with energy production, distribution, and consumption more transparent. When it is not feasible to assess specific externalities comprehensively, the committee will recommend assessment approaches and identify key information needs to inform future assessments. |
dealing with space, time and uncertainty. The committee sought to build on the work of companion studies within the NRC, particularly the AEF and America’s Climate Choices studies.
RELATED STUDIES
National Research Council Studies
With the National Academy of Sciences and National Academy of Engineering having identified energy as a high-priority topic, it has received attention from many NRC committees, both past and current. These studies were relevant to the work of this committee. We briefly discuss two studies here and cite them throughout our report where appropriate.
The AEF’s effort at the NRC was concerned with future technology and its potential for reducing U.S. dependence on oil imports and lowering greenhouse gas emissions, while ensuring that affordable energy will be available to sustain economic growth. The AEF’s task was to critically review recently completed major studies on the potential for change in energy technology and use; compare the studies’ assumptions; analyze the currency and quality of the information used; and assess the relative states of maturity of technologies for potential deployment in the next decade. A secondary focus was on technologies with longer times to deployment.
A study committee and three panels produced an extensive analysis of energy technology options for consideration in an ongoing national dialogue. Collectively, they analyzed advanced coal technologies; nuclear power; renewable energy technologies (such as wind, solar photovoltaic, and geothermal); energy storage and infrastructure technologies; advanced transportation power-train technologies; technologies to improve energy efficiency in residential and commercial buildings, industry, and transportation; and the technical potential for reducing reliance on petroleum-based fuels for transportation. These topics were addressed for three time frames: present-2020, 2020-2035, and beyond 2035.
In response to a request from Congress concerning a related topic, the NRC also launched America’s Climate Choices, a suite of studies designed to inform and guide responses to climate change across the nation (see NAS/NAE/NRC 2009a).
The results of the studies were intended to address the following key questions:
-
What short-term actions could be taken to respond effectively to climate change?
-
What promising long-term strategies, investments, and opportunities could be pursued to respond to climate change?
-
What scientific and technological advances (for example, new observations, improved models, and research priorities) are needed to better understand and respond effectively to climate change?
-
What are the major impediments—for example, practical, institutional, economic, ethical, and intergenerational—to responding effectively to climate change, and what can be done to overcome them?
The AEF and America’s Climate Choices studies are important initiatives that have provided and will continue to provide valuable information on energy technology and policy options for the nation. Indeed, our own study has been informed by the AEF’s analysis of future technology. However, neither of these efforts was designed to focus on the monetary value of energy’s external effects, including climate change.
Prior Externality Studies
The concept of externalities dates at least to the early twentieth century (Pigou 1920) and was discussed extensively in the post-war economics literature (Meade 1952; Scitovsky 1954; Mishan 1965; Arrow 1975). Interest in the externalities of energy production and use gathered momentum in the following decades. Hohmeyer (1988) was one of the more prominent studies during this period. He took a top-down approach in which he estimated the “toxicity weighted” emissions from electricity generation with fossil fuels and then multiplied this fraction by Wicke’s (1986) estimates of total damages from pollution to various end points (such as those on health, forests, and animals). The most prominent study in the United States during this period (Ottinger et al. 1990) used estimates from previous studies that quantified the environmental costs from electric power generation. Results of Niemi et al. (1984, 1987) were among those used by Ottinger et al.; those studies focused on visibility and health effects of airborne particulate matter. Ottinger et al. followed a five-step procedure in using these studies to value environmental damages: emissions, dispersion, exposure, impacts, and damages.
Research in estimating the external costs of energy peaked in the early-to-mid-1990s when public utility commissions in the United States were interested in tilting electric utility investment choices toward sources with lower negative externalities, such as renewable energy. This policy was to be done with an “adder” equal to the marginal damages associated with each type of electricity generation. During this wave of interest, major studies were done by Oak Ridge National Laboratory and Resources for the Future (ORNL/RFF) for the U.S. Department of Energy, by Hagler Bailly for the New York State Energy Research and Development Authority (NYSERDA), by Research Triangle Institute for the State of Wisconsin (one of several states mounting these studies), and by several teams of European research organizations for the European Commission (EC). This latter
study, called ExternE, worked in concert with the ORNL/RFF team to use similar protocols.
Around the same time that work began on the ORNL/RFF and ExternE studies, other studies were completed by the Union of Concerned Scientists (1992); Pearce et al. (1992) in their report to the U.K. Department of Trade and Industry; Triangle Economic Research (1995) for Minnesota; National Economic Research Associates (1993) in its study of Nevada; Regional Economic Research (1991) on California; and Consumer Energy Council of America Research Foundation (1993).
Later, the EC began a companion study on the external costs of transportation as well as other research efforts—the most recent being the New Energy Externalities Development for Sustainability (NEEDS) program—that further refined and developed methodologies extending those developed in ExternE as well as extending those to other energy technologies and study locations. Also in the transportation area, a series of studies were conducted by Greene et al. (1997) and Delucchi (2004), Parry et al. (2007), among several other studies performed since the mid-1990s.
The more notable differences between our committee’s study and previous studies, particularly the major studies done in the early to mid-1990s, are in the different approaches to, and the extent to which, the studies addressed the following:
-
Number of power plants—our study considered almost all coal and natural gas power plants in the country, whereas most other studies focused on a few sites or on plants within a state.
-
Different power-generation options—our study considered fewer technologies than several of the previous larger studies; in particular, our study did not address the nuclear fuel cycle in the detail done in the ORNL/RFF and ExternE studies, which carried out extensive probabilistic risk modeling.
-
The manner in which the dispersion of airborne pollutants and formation of secondary pollutants were modeled—our study used a reduced form approximation of these processes, whereas some previous models used more site-specific, detailed air dispersion and transformation models, albeit for a small number of power plant sites and regulatory scenarios. The early studies also had no or limited modeling and analysis of ozone and fine (2.5 microns or less in diameter) particulate matter formed from the chemical transformation of pollutants emitted by a power plant.
-
Consideration of greenhouse gas emissions and their damages—like all previous externality studies, our study reviewed recent literature rather than undertaking new scientific research; our study reviewed more recent literature than most of the previous studies, although recent studies within the ExternE program used similar literature.
-
Consideration of externalities associated with infrastructure and energy security—of the latter, ORNL/RFF and ExtenE focused only on oil security.
-
The extent to which the entire life cycle of an energy technology (from feedstock through conversion through fuel distribution through energy service) was analyzed—the ways in which the different technology fuel cycles were analyzed—the ORNL/RFF, ExternE, and our study considered life-cycle impacts, whereas most other studies focused on electricity generation or use of vehicles in transportation and not on the upstream activities in the life cycle.
-
The extent that externalities, other than those associated with electricity generation, were addressed in the same study (that is, transportation and energy used for heat).
With some exceptions, the “adders” studies of the early and mid-1990s took a place-based approach to damage estimation of energy. They would posit the construction of a new electricity-generating plant of a particular type at a given location. Each study considered a small number of alternative locations for each plant, generally from two to five. In those studies, the different results calculated for the different plants would reflect the influence of the specific location of the plant on the magnitude of the damages. In contrast, our study calculates the health-related and some of the environmental damages for most of the power plants in the United States and estimates the damages from each. In this respect, it is similar to the U.S. Environmental Protection Agency’s regulatory impact analyses that use the BenMAP model and to the efforts of Muller and Mendelsohn (2007), which, although not studies of externalities per se, are comprehensive in their level of spatial resolution, such as addressing the specific location of all power-plant emissions.
The ORNL/RFF and ExternE studies included relatively detailed engineering descriptions of the technologies of the power plants, whereas our study and most other studies focused on estimates of emissions from power plants and not on the underlying technologies.
In estimating the health and environmental damages, the ORNL/RFF and ExternE studies used different detailed models to predict the dispersion of primary pollutants from the power plants and the atmospheric formation of secondary pollutants, specifically ozone and fine particulate matter. Studies of externalities associated with greenhouse gas emissions generally either focus exclusively on these emissions and the associated climate change, as exemplified by the authoritative reports of the Intergovernmental Panel on Climate Change, or focus on other pollutants. Our study, on the other hand, provides a range of quantitative estimates of the damages from climate change in monetary terms. The previous externality studies either
do not include this important issue in their analysis or, as in the case of the ORNL/RFF and ExternE studies, draw on a more dated and limited scientific literature in this area.
Externalities associated with infrastructure and energy security are usually not addressed in other studies. Our report considers electric grid externalities, infrastructure vulnerability to attacks and accidents, and national security. The ORNL/RFF study provided estimates of damages from dependence on foreign oil, and other studies focused on this issue provided updated estimates. However, as discussed in our report, although damages might result from global dependence on oil in a cartel-dominated market, such damages are not considered externalities.
The ORNL/RFF and ExternE’s consideration of damages from different parts of the life cycle, for example, coal mining, sets them apart from most other studies, which do not consider externalities on a life-cycle basis. Several studies have estimated life-cycle emissions of some fuels currently, or prospectively, used in ground transportation; these studies did not attempt to estimate the impacts and associated externalities of these emissions. Our study, on the other hand, takes an energy life-cycle approach somewhat similar to the ORNL/RFF and ExternE studies, but with more updated considerations and data.
Although many studies have addressed different aspects of the externalities from energy production, distribution, or use to varying degrees, they have focused on one type of externality (such as health effects), or one particular sector (usually electricity generation or transportation). Also, they generally focused on one part of the energy cycle. In contrast, our study has a relatively comprehensive scope that includes all types of externalities from energy life cycles of both electricity and transportation, as well as from production and use of energy for heat in residential, commercial, and industrial sectors.
DEFINING AND MEASURING EXTERNALITIES
Defining Externalities
External effects or “externalities” are important because failure to account for them can result in distortions in making decisions and in reductions in the welfare of some of society’s members.
An externality, which can be positive or negative, is an activity of one agent (for example, an individual or an organization, such as a company) that affects the well-being of another agent and occurs outside the market mechanism. In the absence of government intervention, externalities associated with energy production and use are generally not taken into account in decision making. Box 1-2 provides definitions of the technical terms used in
BOX 1-2 Definitions of Key Terms Much of the nomenclature for the key terms is taken from the damage function approach, which has been the standard approach to examine the costs and benefits of environmental regulations, required by OMB (NRC 2002a). This approach begins with some burden, say emissions, which ultimately has some physical effect; this effect is then monetized and termed damage. The monetary value of reductions in burdens is termed benefits (the opposite of damages). Burdens: Externalities from economic activities are always by-products of those activities, some of which are useful and some of which cause health and environmental effects, for example. The by-products themselves are termed burdens. Emissions of air pollutants and discharges of pollutants into a river are examples of burdens. Effects and Impacts: These burdens have a real effect in the environment, that is, they have a physical component that affects health, damages ecosystems or reduces visibility, for example. Sometimes, as with energy security, the physical component is not directly present. In any event, these physical effects are termed effects or impacts. Damages: Damages are the monetary value of the physical effects, in its simplest form calculated by multiplying the quantity of physical effects of interest by a monetary value for that effect. This monetary value represents, ideally, the population average of the maximum willingness to pay for a unit improvement in this physical metric. That is, it reflects the preferences people have for reducing this physical effect, given their income and wealth. It is analogous to the price people are willing to pay for a product for sale in a market. Benefits are the opposite of damages. |
this report that bear on externalities. Some examples of externalities using these terms are presented below. An additional illustration is presented in Appendix B.
Example 1. A coal-fired electricity-generating plant, which is in compliance with current environmental regulations, releases various pollutants to the atmosphere that adversely affect the health of residents. The pollution released by the plant is an example of a negative externality because it contributes to health problems for residents. The damage from this pollution is an additional cost of production to society (a “social cost”). If these social costs were not adequately taken into account in selecting the plant’s site or the air pollution control technology that it uses, the true costs of the plant have not have been reflected in these decisions.
Example 2. Many thermal power plants use water for cooling; therefore, they emit heated effluent, which sometimes benefits anglers because fish in cold regions are attracted to the warmer water. Therefore, the fishing is better in the effluent plume. This is an example of a positive externality.
Total versus Average versus Marginal Damages: To be most helpful for policy, we want to estimate marginal damages and compare these to marginal costs to reduce these damages. The marginal damage is the damage that arises from the last unit of emissions or other type of burden. In many cases, marginal damages are constant over the relevant range of emissions. That is, the damage from the last unit is no different than the damage from the first unit of emissions. But, in some cases, say for pollutants that accumulate in the environment, marginal damages grow with more emissions. In any event, for policy purposes, if the marginal damages from the last unit of emissions exceed the marginal costs from eliminating that unit, then it would benefit society to eliminate that last unit, since the damage prevented would exceed the cost of preventing that damage. Total damages, in contrast, are the sum of marginal damages for all units of emissions. Average damage is the total damage divided by the number of units of emissions or other burdens in question. Average and marginal damages may equal one another under certain conditions, but in general they are different. In this report, sometimes we assume that they are equal because it is easier to calculate average rather than marginal damages and actual differences are expected to generally be within error margins. Externalities: An externality, which can be positive or negative, is an activity of one agent (that is, an individual or an organization like a company) that affects the wellbeing of another agent and occurs outside the market mechanism. In the absence of government intervention, externalities associated with energy production are generally not taken into account in decision making. |
The improved angling is a societal benefit that probably was not reflected in the utility’s decisions about where to site the plant and effluent. This societal benefit does not take into account any other ecosystem changes, which might or might not be seen as beneficial.
Example 3. A company is building a new coal-fired power plant in a small community and hires a large number of construction workers. The increase in demand for construction workers drives up the local wage rate and adversely affects homeowners who wish to hire workers to remodel their homes. The price of their remodeling projects has gone up with the increase in wages. This is not an externality, however, because the activity of one agent (the company building the power plant) affects other agents (homeowners wishing to remodel their homes) through a market mechanism—the labor market. The company takes the increase in wages into account because it must also pay the higher wages to attract construction workers.
Example 4. Farmers respond to a demand for corn-based ethanol by diverting land from food production to fuel production. The reduction in
the supply of feed corn and other grains drives up the prices of grains and meat, thereby making consumers worse off. This is not an externality since the activity of one agent (fuel buyers bidding up the price of corn for ethanol production) affects other agents (the food-buying public) through the markets for corn and other food products.
Example 5. Workers in high-risk occupations receive a higher wage than workers doing similar tasks in jobs with lower risks. This is not an externality because those bearing the risks are freely choosing within a market to accept this risk and are compensated for the risks they face through higher wages. Increased costs faced by a firm do not by themselves indicate whether the firm’s activities are an externality. For example, electricity-generating plants participating in the Acid Rain Program of the U.S. Clean Air Act face higher costs because they must surrender valuable permits for each ton of sulfur dioxide (SO2) emitted. This higher cost is the result of a government program to reduce the externality associated with acid rain. In the case of elevated costs to compensate for high-risk jobs, no government policy is involved in addressing the risks faced by employees due to the nature of the work they are offered.
Externalities matter because, when they are not accounted for, they can lead to a lower quality of life for at least some members of society. For example, suppose that the power plant in the first example has access to technology that, at a cost of $40/ton, can cut its emissions by 10 tons. Suppose further that the full cost of the effects that residents suffer (for example, health and psychological costs) is $50/ton. If the plant were to install the technology, total social welfare would increase—the additional cost to the plant would be $400, but the “savings” to the residents (that is, the reduction in adverse effects they suffer) would be $500. Human well-being would be increased by this change. However, if the externality had not been accounted for in the plant’s decisions, aggregate well-being of all members of society would be lowered.
It is important to distinguish true negative externalities from unfortunate market signals (such as higher prices of food) that hurt some members of society but are not externalities. The reason for this distinction is that, in the case of a true externality, the possible well-being of society can be raised by accounting for it—the “pie” that represents the value of society’s goods, services, and related intangibles is enlarged. If it is not a true externality, market intervention cannot alter the size of the pie but can only reallocate it.
Note the following additional points about externalities:
-
The agents that produce externalities can be organizations or individuals. For instance, a restaurant diner who smokes an after-dinner
-
cigarette (besides breaking the law in most states) creates an externality for others in the restaurant.
-
The activities that produce negative externalities usually also produce benefits for someone in society. The electricity that is produced by the coal-fired power plant provides value to the consumers who purchase that electricity. The externalities arise from the side-effects of that benefit-producing activity that are not reflected in its market price.
How Externalities Are Characterized in This Study
Most of the externalities associated with the production and consumption of energy have been addressed, or corrected, to some degree, through public policies. Coal mining and oil and gas extraction are subject to federal, state, and local regulations that are intended to limit the environmental damages from fuel extraction. Air pollution emissions by power plants are regulated under the Clean Air Act, and tailpipe emissions from motor vehicles are regulated at the federal and state levels. Indeed, regulations designed to correct externalities have substantially reduced their magnitude over the past 30 years. What the committee evaluates in this study are the externalities that remain in 2005—and the externalities that are predicted to remain in 2030—after such regulations have been implemented.
To make clear what we evaluate in this study, consider Figure 1-1, which shows the marginal damage associated with SO2 emissions and the marginal cost of emitting SO2 for a hypothetical power plant in a particular year. According to the graph, the damage from emitting each additional ton of SO2 is $1,000. The cost of emitting another ton of SO2 declines as more is emitted—equivalently, the marginal cost of reducing SO2 emissions (moving from right to left on the horizontal axis) increases as less SO2 is emitted. If Firm 1 (the hypothetical power plant) in 2005 is emitting E1 tons of SO2, the external damages that we quantify equal the shaded area in Figure 1-1—that is, we quantify the total damages associated with the firm’s current level of emissions.1 If the firm were emitting E* of SO2, the external damages that we evaluate in the study would correspond to the rectangle 0ABE*. We also express these damages per kilowatt-hour of electricity produced and quantify the marginal damage of each ton of SO2 produced by the plant for a specific year.
It is important to note that, from the viewpoint of economic efficiency,
1 |
We calculate aggregated damages by estimating the damages per ton of SO2 (the horizontal line in Figure 1-1) and multiplying by the number of tons of SO2 emitted in 2005, to which we add similar calculations for NOx and directly emitted PM. We therefore calculate the marginal damage per ton of SO2 (and per ton of NOx and PM) at each plant, which are reported in Chapter 2. |

FIGURE 1-1 Marginal damage associated with SO2 emissions in a year (x-axis) and the marginal cost of emitting SO2 in a year (y-axis) for a hypothetical power plant (Firm 1) emitting SO2. E1 = amount of SO2 (tons) emitted by Firm 1; E* = economically optimal level of SO2 emissions; MC1 = marginal cost for Firm 1. Damages of emitting each additional ton of SO2 are assumed to be constant.
the externalities that we characterize may be too large or too small. The economically optimal level of SO2 emissions occurs at E* where the cost of reducing the last ton of SO2 equals the corresponding reduction in damages. Even at the optimal point, damages occur—an externality remains, even though emissions are at an economically optimal level.2
What then is the significance of the externalities presented in this study? As is clear from the diagram, whether emissions should be reduced or increased from the viewpoint of economic efficiency depends on the current level of emissions and the cost of reducing them; it cannot be determined from the size of total damages alone. However, evaluating economic efficiency requires information about the costs of abating SO2, which are outside the scope of this study. This does not mean that information about total damages is without value on its own: Plants with large total damages
2 |
Some economists might say that there is no externality if emissions are at the optimal level (E* in Figure 1-1). However, the committee follows Baumol and Oates (1988) in saying that an externality exists, even though emissions are at an optimal level. |
should likely be subject to a benefit-cost analysis of pollution control measures to see whether further pollution control is warranted.
Economic theory also suggests efficient methods for regulating the externalities associated with air pollution. For example, the first best solution to internalizing the damages in Figure 1-1 could be achieved by imposing a tax on SO2 emissions equal to the marginal damage that the emissions impose—a tax equal to the height of the marginal damage curve. This solution could also be achieved by a pollution permit market in which firms traded rights to pollute denominated in damage terms (Roumasset and Smith 1990; Hung and Shaw 2005).3 In either case, a firm would have an incentive to reduce its rate of SO2 emissions to E*.4 Information on the marginal damages associated with various pollutants, which we quantify for fossil-fueled power plants, is relevant to the efficient regulation of air pollution externalities.5
Nonexternality Market Distortions and Impacts
In markets related to energy production and consumption, many other distortions occur that create opportunities for improvement of social welfare but that are not externalities. Because these distortions are outside the purview of this study, the committee touches only briefly on them here, largely to be clear that we do not attempt to identify and quantify the social costs associated with those distortions and to recognize that the magnitude of externalities can be directly affected by the presence of these distortions.
One form of market distortion that affects energy markets is the presence of market power—in the extreme, a single supplier of energy (monopoly) or a single buyer (monopsony). In such cases, a firm with market power can affect the price and quantity traded to its advantage and impose costs on others that exceed its gains. Cartels, such as OPEC, or large purchasers of oil, such as the United States, can exhibit market power.
Another form of market distortion that affects energy markets is the presence of taxes or subsidies (“tax breaks”) that do not correct externali-
ties but are imposed to raise revenue, provide support to an industry, or serve some other purpose. Of chief concern for this study is that these subsidies can affect the amount of an externality generated by an industry. For example, subsidies for oil exploration have encouraged the expansion of the petroleum industry, thereby increasing the magnitude of the externalities generated by the production and consumption of petroleum.
Information asymmetries and public goods are two additional cases of market failure that could affect energy markets. In this study, we quantify to the extent possible the noninternalized externalities conditional on the existing set of market regulations, taxes, subsidies, and market distortions from all sources.
In addition to market distortions that are not externalities, there are impacts of energy production and use that may be of public concern but that are not externalities. For example, as discussed earlier, increased corn prices due to the production of biofuels do not represent an externality as they result from the proper functioning of a market. This is not to say that higher corn prices are unimportant or that they should not be the subject of public concern and policy. However, there is no market failure to correct, nor is there an externality to internalize. Another important example is the distribution of social costs across space, time, or different population groups. Such distributional issues may be of great concern to policy makers, but they do not represent externalities.
In this study, we have restricted our attention to externalities, but we do point out some nonexternal impacts when relevant. For example, occupational injuries in coal mining and oil and natural gas extraction do not qualify as externalities according to our definition; however, there is interest in many quarters in documenting the magnitude of these impacts. Thus, we quantify (but do not monetize) them below.
SELECTING ENERGY SOURCES AND USES FOR THIS STUDY
The committee’s task was the evaluation of externalities “associated with the production, distribution and consumption of energy from various selected sources.” Studying selected sources was necessary because it would have been infeasible to evaluate the entire energy system given the time and resources available to the committee. In selecting the sources for study, the committee was careful to include major elements of the energy system and the most significant externalities.
To create a basis for the committee’s selection of energy sources and their end-use services, we started with the general framework shown in Figure 1-2. Energy sources depicted in the figure are fossil fuels (such as coal, petroleum, and natural gas), nuclear, and the various “renewables” (such as biomass, solar, and wind). Also shown are the various forms of
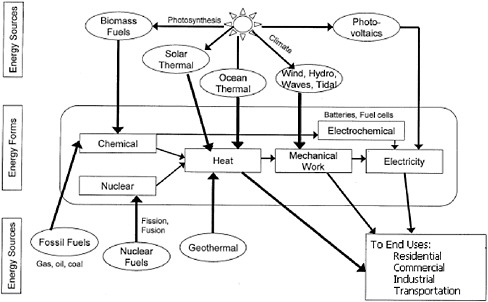
FIGURE 1-2 Sources and forms of energy that provide the ability to do useful work. SOURCE: Tester et al. 2005. Reprinted with permission; copyright 2006, MIT Press.
energy that these sources represent or into which they are converted for use by industrial and residential users and in transportation. For example, coal is combusted in electric power plants to produce heat that is used to turn turbines (mechanical work) that turn generators that produce electricity that is transmitted to houses and factories.
Some energy sources, such as solar, produce heat directly without a combustion step, and others, such as wind, produce mechanical work directly. Furthermore, heat is, of course, used directly (in homes, for example) from, say, natural gas, without further conversion into mechanical work or electricity. Although they are not depicted in the diagram, it is worth keeping in mind that there are energy losses associated with the conversion, movement, and use of energy. In general, the more steps in the process, the less efficient the use of energy.
Figure 1-3 shows energy flows from primary energy sources to end uses in the United States in 2007. Total U.S. energy usage that year amounted to 101.5 quadrillion British thermal units (quads), so the numbers shown in the figure also approximately correspond to percentages. We note that electricity is an intermediate form of energy. From the perspective of the impacts of energy use in the United States, electric power is especially

FIGURE 1-3 Energy flows in the U.S. economy, 2007. An illustration of energy movement from primary sources (boxes on the left) to consumption by end-use sectors (residential, commercial, industrial, and transportation) (boxes on the right). SOURCE: Prepared by Lawrence Livermore National Laboratory and the Department of Energy (NAS/NAE/NRC 2009a, p. 17).
important, even though it is not an energy source or end use per se. The losses associated with energy transformation and transport are also shown as “rejected energy” or loss of useful energy. The amount of rejected energy (58.47 quads) in 2007 exceeded the amount of energy that provided energy services (43.04 quads).
The contribution percentage of each energy source to total U.S. energy consumption in 2007 is shown in Figure 1-4. Primary energy use and delivered energy use by sectors are shown in Figures 1-5 and 1-6, respectively. In Figure 1-5, the shaded regions in the end-use and electric-power bars show how much each source contributed to the total U.S. energy use of 101.5 quads. For example, petroleum use for transportation was approximately 28 quads in 2007. Figure 1-5 also shows that, of the 101.6 quads used in 2007, 40.6 quads were in the form of electricity.
In Figure 1-6, each end-use sector shows the total 2007 energy use, whether from electricity or from the “primary” energy sources in that sector. For example, the figure shows that industrial end users used 33.4 quads in 2007, 12.0 quads of which were in the form of electricity.
Figures 1-3, 1-4, 1-5, and 1-6 contain several notable facts about U.S. energy production and use. In addition to the overwhelming dependence of transportation on petroleum, noted above, we also see, for example, that
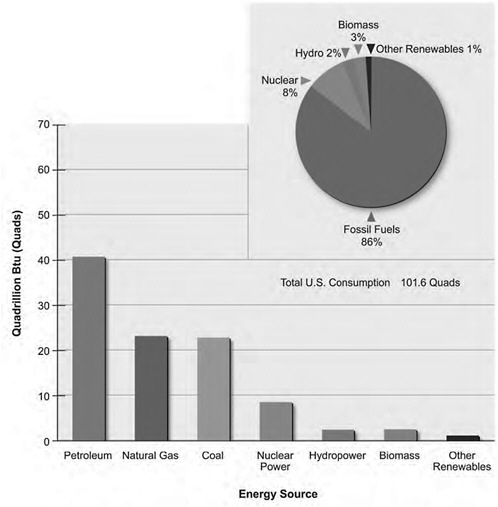
FIGURE 1-4 U.S. energy consumption by energy source in 2007. SOURCE: Data from EIA 2008a, Table 2.1a.
the vast majority of coal use in the United States is for electricity generation and that renewables and nuclear energy each represent relatively small fractions of total energy use.
Table 1-1 shows the sources and end uses selected for the analysis of external effects in this report. The energy sources are arrayed as rows. (Note that we have listed each of the renewables separately.) The end uses are shown as columns; here, for convenience, we have listed electricity production as an end use, and we have focused our attention in the industrial sector and commercial/residential sectors (buildings) on their use of energy for heating.
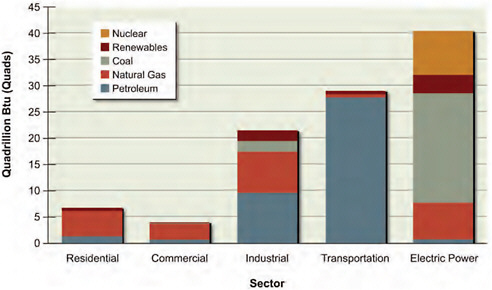
FIGURE 1-5 U.S. consumption of energy by sector and fuel type in 2007. Natural gas is the major fuel type for the residential and commercial sectors. Petroleum and natural gas are the major fuel types for the industrial sector. Petroleum is by far the major fuel type for the transportation sector. For electric power, coal is the major fuel type, followed by natural gas and nuclear power. Energy consumed by the electric power sector is used to produce electricity consumed by the end-use sectors. SOURCE: EIA 2008b, in NAS/NAE/NRC 2009a, p. 22, Figure 1.8.
The committee discussed every cell in Table 1-1 at length and selected the shaded cells for evaluation. Given the available time and resources, we chose those cells that represented the largest portions of the U.S. energy system or those that represented energy sources with substantial increases (>20%) in consumption over the past 5 years (2002-2007). Thus, coal for electricity production and petroleum for transportation, which are the two largest single components of the U.S. energy system, were obvious choices. Natural gas is an important fuel, comparable to coal as a source. We included its use for electricity production and space heating in buildings. Wind, biomass, and solar were also included because of increased consumption in recent years.
The committee also included cells that represented sources and uses of particular current policy interest or that are expected to grow in significance (such as nuclear energy for electricity production). The base year for the committee’s analyses was 2005 (or the nearest year for which data were available). We chose a future year to gain insight into the impact of future technologies on energy externalities. We chose 2030 for this purpose so as to be consistent with and leverage the work of the AEF.
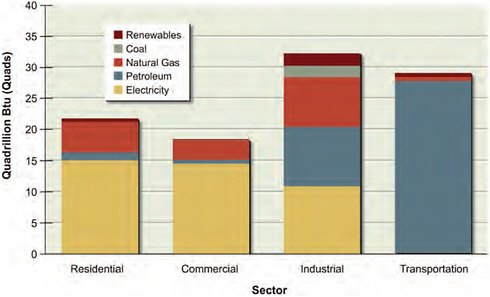
FIGURE 1-6 U.S. delivered energy consumption by end-use sector in 2007. Electricity predominates in the residential and commercial sectors. Electricity, petroleum, and natural gas are the main forms of delivered energy for industry. Petroleum is by far the major fuel type for the transportation sector. SOURCE: EIA 2008b, in NAS/NAE/NRC 2009a, p. 22, Figure 1.9.
Identification of significant alternative energy technologies that are being considered for implementation before 2030 was guided by the AEF reports and other sources. Those that were evaluated are already in various stages of development and commercialization, so some information on potential externalities was available. Other technologies that are still in research and early demonstration phases may be available for commercialization by 2030, but the committee decided that credible evaluation of externalities would be premature until more was known about actual performance. The future technologies evaluated for the 2030 time-frame are as follows:
-
Electricity Sector (Chapter 2): Nuclear power impacts are included in the present electricity-generation portfolio and will apply to continuing or expanding use of this source. Biomass, wind, and solar photovoltaics (PV) are already deployed to some extent in the present electricity production portfolio. In 2030, all of these technologies may be advanced and constitute a more important part of the electricity mix, so it is important to assess their externalities—both positive and negative. The AEF panel report on Electricity from Renewables (NAS/NAE/NRC 2009b) states that a “rea-
TABLE 1-1 Committee Study Approach for Energy Sources and Consumption Sectorsa
|
-
sonable target” for 2020 is to have nonhydropower renewables as 10% of the generation mix. Most of this is expected to come from wind, as solar PV technologies still face challenges of costs and technology development that limit likely deployment to a few percent on this time scale. Not evaluated were the following: hydropower (about 2.5% of the sector and unlikely to grow much); geothermal (about 0.3% of present use and unlikely to grow much until enhanced geothermal system technologies, for example, deep-heat mining, are improved); and fossil-fueled power plants with carbon capture and storage (CCS), which seem technically feasible but still are far from large-scale implementation and will require new infrastructure for carbon dioxide transportation to storage sites of proven integrity. The addition of significant amounts of variable and nondispatchable electricity sources (solar, wind) also presents challenges to the operability and reliability of the electric power grid, which has aging systems that need upgrading. These issues are considered in more detail in the AEF report Electricity from Renewables: Status, Prospects, and Impediments (NAS/NAE/NRC 2009b)
-
and were not a major focus in this study, but we do discuss them in the context of “network externalities” in Chapter 6.
-
Transportation Sector (Chapter 3): In the 2030 time frame, petroleum is still likely to be the major transportation fuel. There may be increased use of ethanol as a blend with gasoline or as a pure fuel. The analysis considers corn, cellulosic ethanol, cellulosic synthetic gasoline, and other feedstocks as biofuel options. Electric cars and plug-in hybrids are discussed and tied to externalities from the electricity sector analyses. Compressed natural gas is also an alternative fuel that may find use in both light- and heavy-duty vehicles. Some of the gasoline in use today comes from energy intensive production of Canadian oil sands; synthetic gasoline (or diesel) also can be made from coal, natural gas, or biomass. The AEF panel assessing possible options for producing alternative liquid transportation fuels concluded that CCS would likely be needed to reduce GHG emissions resulting from production of liquid fuels from coal (NAS/NAE/NRC 2009c). CCS systems are not yet well enough evolved for any sort of a meaningful externalities analysis.6 The committee evaluated hydrogen (made from fossil fuels or electrolysis of water) to some extent, as a possible conveyor of energy for transportation in the 2030 time frame, although its use will be limited because of the considerable need to develop storage and infrastructure technologies. Although aviation jet fuel is likely to remain petroleum based until alternative synthetic fuels are developed, there are some special externalities associated with aviation that will be addressed separately with regard to climate change (Chapter 5).
-
Energy for Heat (Chapter 4): Consumption of energy for the production of heat in the buildings sector mostly involves natural gas, along with a little petroleum. The primary change for 2030 was assumed to be efficiency gains due, for example, to changes in building design and construction. Much of the information about potential efficiency gains was based on the AEF efficiency panel report, Real Prospects for Energy Efficiency in the United States (NAS/NAE/NRC 2009d). Because of its diversity and complexity, the industrial sector was discussed from a general perspective.
FRAMEWORK FOR EVALUATING EXTERNAL EFFECTS
The Life Cycle of Energy Use
When considering the external effects of using energy, people often focus on the end use—for example, the air pollution from burning gasoline
in an automobile. In fact, identifying energy’s external effects requires a broader view that reflects energy’s entire life cycle, from extraction of the energy source as it is found in nature through conversion, transportation, and transmission to its point of use and then to the ultimate fate of waste products from that use. Thus, the committee adopted life-cycle assessment (LCA) as its approach to identifying the external effects of energy.
There are two general types of LCA: process-analysis-based LCA and economic input-output (EIO) analysis-based LCA. Process-based LCA considers all inputs (including raw materials, energy, and water) and all outputs (including air emissions, water discharges, and noise of included processes associated with all life-cycle stages of a product or service). EIO-LCA helps address boundary-selection problems and data intensity by creating a consistent analytical framework for the economy of an area based on government-compiled input-output tables of commodity production and use coupled with material and energy use and emission and waste factors per monetary unit of economic output. It may not provide the level of detail of a process-based LCA. An important metric of the system studied is referred to as a functional unit (for example, vehicle miles traveled can be used as the functional unit for LCAs related to transportation). Because of the significant variability in assumptions, boundaries, and approaches, comparisons across different assessments must be done with caution (see discussions, especially in Appendix E, in NAS/NAE/NRC 2009b).
Figure 1-7 depicts the major elements of energy use from an LCA perspective. Taking the use of domestic petroleum for automobiles as an
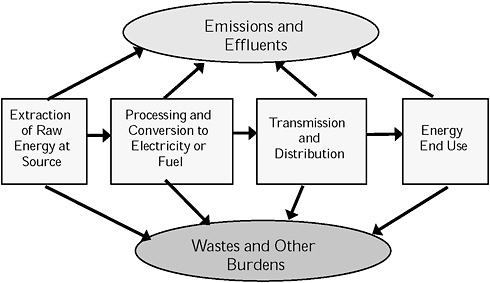
FIGURE 1-7 Life-cycle analysis for energy use.
example, the first box in Figure 1-7 would include the exploration and drilling for oil and sending it via pipeline, tanker, or truck to a refinery; the second box would include refining to produce gasoline; the third box would include transport of gasoline by pipeline and trucks to service stations; and the fourth box would include the burning of gasoline in automobiles. Each stage in the life cycle produces impacts, and all of them should be considered in estimating the external effects of energy use. Staying with the gas-powered auto as an example, the externalities associated with burning a gallon of gasoline are composed of the external effects of all the “upstream” activities necessary to produce and deliver that gallon of gas to the pump, as well as the direct impacts of burning the gasoline in the car. Further, on the basis of vehicle miles traveled, there are externalities associated with the car itself—the extraction and production of materials used in the manufacture of the car, the sales distribution network, the maintenance required during the life of the car, and the ultimate disposal of the car.
Energy efficiency and energy conservation are important aspects of overall energy policy, and often are considered as being equivalent to increasing the energy supply. Energy conservation refers to reducing energy use by reducing services that require energy; examples include lowering indoor temperatures in winter and raising them in summer to reduce the use of energy, and walking or riding a bicycle to reduce the use of gasoline. Energy efficiency refers to using less energy to achieve the same level of energy-based service; examples include using compact fluorescent light bulbs instead of incandescent ones to achieve the same ambient light level with less electricity and using more efficient internal combustion engines or hybrid systems to achieve the same transportation capacity with less fuel.
Identifying and Quantifying Burdens, Impacts, and Damages
With the life-cycle stages identified, determining the externalities of energy required the committee to identify the burdens, impacts, and damages associated with each stage. The methodology followed what is termed the damage-function approach (Jolliet et al. 2004). The burdens come in many forms (for example, air emissions, liquid discharges, and solid wastes); they move through all media (air, water, and land); and they have a range of effects or impacts (on human health, natural ecosystems, and the built environment). All of these factors must be accounted for in producing a complete estimate of damages, although, as will be seen, for many energy uses some impacts and damages are far more important than others. Furthermore, some impacts are not externalities (see Box 1-2). The committee made judgments about what damages are likely to be externalities or are widely considered to be externalities (such as damages associated with energy security) and focused its work on those.
Table 1-2 shows impacts at each stage of the life cycle for generating electricity from coal. These impacts are all externalities, with the exception of occupational injuries among coal miners, which are viewed as a job characteristic that is traded in labor markets. The table is intended to be illustrative; Chapter 2 provides a more thorough discussion of the external effects of electricity generation.
Some impacts are direct, easy to understand, and often well-supported by data. The deaths and injuries suffered by coal miners, although not externalities, represent an example of this. Other impacts, such as the alterations in water availability or ecosystems due to climate change, are the result of very complicated physical, chemical, and biological processes about which there is great uncertainty.
In this study, given the constraints on time and resources, the committee relied on past work in the identification of impacts and their damages. We did not attempt to develop new methods for estimating impacts and damages, but we did identify areas where additional research would be particularly valuable. It is important to realize that estimating most of these impacts and damages is a several-step process based on many assumptions; this is true for even relatively well-understood impacts. Consider one of the
TABLE 1-2 Illustrative Impacts of Producing Electricity from Coal
|
Human Health |
Ecosystems |
Security and Infrastructure |
Coal Mining |
Coal miners’ mortality and morbidity |
Land disturbance river alteration, acid mine drainage |
|
Transportation of coal to power plants |
Death and injury from accidents |
Vegetation damage from air pollution |
Load on transportation systems |
Burning of coal |
Mortality and morbidity from air pollution |
Ecosystem effects from cooling Water discharges Ecological changes from climate change |
Degradation of building materials Agricultural shifts and coastal community impacts due to climate change |
Disposal of waste |
Health effects of heavy metals in ash and other waste |
Ecosystems effects of ash and other wastes |
|
Transmission of electricity |
|
Disturbance of ecosystems by utility towers and rights of way |
Vulnerability of transmission system to attack or disaster |
boxes in Table 1-2: human health effects of air pollution from coal-fired power plants. Arriving at an estimate requires
-
Estimate burdens: air-pollutant emissions (which depend, among other things, on the particular coal that is used, the boiler technology, and the pollution-control technology).
-
Estimate the ambient concentrations of air pollutants (which depend, among other things, on the height of the exhaust stack, the location of the plant, and prevailing wind patterns).
-
Estimate the exposure of people to air pollutants (which depends, among other things, on where people live and how much time they spend outside).
-
Estimate effects or impacts: the health consequences of the exposures (which depend, among other things, on the age and health status of the exposed population and their life styles, for example, whether they smoke).
-
Estimate damages: the value of avoiding these impacts to society (which depends, among other things, on the incomes of individuals and the seriousness of the impact, as judged by individuals)
This emission → ambient concentration → exposure → impact → damage estimation process is generalizable; it underlies each box in Table 1-2 and the estimation of most of the damages considered in this study. Security of energy supplies and network externalities, both discussed in Chapter 6, do not fit as neatly into this framework as virtually everything else does.
A noted, many assumptions must be made. Many of the assumptions are specific to the case at hand, and we make clear in the context of each analysis what the key assumptions are. One cross-cutting issue is the distribution of impacts and damages, both spatially and within populations. The issue is discussed later in this chapter.
Evaluating Impacts
Evaluating damages requires an estimation of the impacts—the tangible manifestations of the burdens of energy use. Thus, we have to express impacts in tangible terms and associate with each impact a metric or measure.
In Table 1-2, the impacts are all physical or biological. Describing some of them is fairly straightforward—for example, death or injury from transportation accidents can be associated with a metric. For example, a metric for the human health effects from coal transportation is the annual deaths from transportation accidents. Another metric is the number of injuries from transportation accidents. Both are meaningful, easily understood
measures or indicators of the impact of interest, and data are available for quantifying them.
Other impacts are not nearly so straightforward. Virtually all of the ecosystem impacts—no matter their cause—present a real challenge for evaluation. One could say that the overarching concern is ecosystem health, but this is not a useful metric: How would one define it or quantify it? We could use physical, chemical, or biological surrogates—for example, changes in water temperature, the concentrations of key chemical constituents in water, and biological productivity. Each of these is well-defined and measurable, but it is arguable whether they would adequately reflect changes in ecosystem health, individually or collectively (see EPASAB 2009).
A potential source of complexity in impact assessment is cumulative effects, which can be important but are often inadequately assessed. Our discussion here largely follows NRC (2003a).
Concern about cumulative effects started in a formal sense with the passage of the National Environmental Policy Act (NEPA) of 1969, motivated by the environmental effects of multiple electric power plants. In 1978, the President’s Council on Environmental Quality (CEQ) promulgated regulations for implementing NEPA (40 CFR Parts 1500-1508 [1978]), which defined a cumulative effect as “the incremental impact of the action when added to other past, present, and reasonably foreseeable future actions. … Cumulative impacts can result from individually minor but collectively significant actions taking place over a period of time.” Thus, a single power plant might have only minor, or acceptable, impacts on, say, an estuary but the effects of multiple plants might be substantial and qualitatively different from the effects of only one plant. In other words, the effects can accumulate. Similarly, some of the effects of smokestack emissions from power plants can interact with those of automobile and industrial emissions; in addition, effects can accumulate over time. As NRC (2003a) pointed out, effects can be synergistic or antagonistic (in other words, they can be greater or smaller than the sum of their parts), and they can have thresholds.
Indeed, thresholds are an important complication in measuring impacts, especially for some environmental effects. The concern is not only with, say, increased water temperatures but also with temperatures above a certain level at which especially significant biological impacts occur, for example, massive fish kills.
Many threshold effects, like water temperatures, are relatively well-understood and predictable based on scientific knowledge. Others are more controversial due to the uncertainty of the underlying science and the economic and other consequences of dealing with the impact. For example, there has been a long standing disagreement over whether there is a nonzero level of exposure to radioactivity that is safe for humans. A more recent example relates to climate change and the potential for relatively rapid and
perhaps irreversible shifts in certain subsystems of the earth, producing, for example, accelerated melting of ice sheets and caps.
Because the ultimate concern is human health and the environment (or more, generally, social welfare), these threshold effects are important. Water temperature can be measured reliably, but the temperature is not what people really care about; it is how life-forms respond to that temperature. If the two are proportional responses, then a physical measure, such as water temperature, can be a useful surrogate metric. When the proportionality breaks down, as it does when there is a sharp threshold, the surrogate loses its utility.
Beyond cumulative effects, thresholds, and other physical complexities, some impacts are very hard to quantify because they are highly subjective. Aesthetics is a good example of a factor whose quantification is highly uncertain. For example, harvesting trees in a forest affects the aesthetic appearance of the forest. One might consider the number of trees removed as a metric. Of course, the problem with such a metric is that it is not only the number of trees; it is also which trees.
Qualitative impacts present a special challenge to communicate, particularly to do so in a way that decision makers take them into account. The adage, “You can’t manage what you can’t measure,” might be dangerous or, at least, limiting if the absence of quantification is taken to mean a value of zero (see the discussion of uncertainty at the end of this chapter).
Table 1-3 summarizes impact pathways and associated effects evaluated in an ExternE study on electricity and transportation.
Damage Estimation: Monetizing Impacts
It is relatively straightforward to monetize goods that are routinely traded in markets, as the market prices give direct information about their monetary worth. Thus, if a family is willing to purchase salmon at the market price, then the value of the salmon must be at least as great as the purchase price, otherwise the family would not have been willing to give up the other items its members could have purchased with that money. The market price gives important information about how much this family is willing to trade off other items to have salmon for dinner. However, many of the externalities that we are interested in for this report do not trade in markets, so information on people’s preferences is not readily available. Nonetheless, such “nonmarket” goods may have as much or more value to people as goods that do trade in markets (that is, if required, they would be willing to give up a lot to have them).
The main goal in monetizing the impacts of externalities is to place externalities on equal footing with other goods and services. When decision makers must decide, for example, whether to tax an externality, increase
TABLE 1-3 Illustrative Impact Categories Pathwaysa
Impact Category |
Pollutant/Burden |
Effects |
Human health: mortality |
PM, SO2 |
Reduction in life expectancy |
|
Benzene, Benzo[a]pyrene 1,3-butadiene Diesel particles |
Cancers |
|
Noise |
Loss of amenity, impact on health |
|
Accident risk |
Fatality risk from traffic and workplace accidents |
Human health: morbidity |
PM, O3, SO2 PM, O3 PM, CO |
Respiratory hospital admissions Restricted activity days Congestive heart failure |
|
Benzene, Benzo[a]pyrene 1,3-butadiene Diesel particles |
Cancer risk (nonfatal) |
|
PM |
Cerebrovascular hospital admissions Cases of chronic bronchitis Cases of chronic cough in children Cough in asthmatics Lower respiratory symptoms |
|
O3 |
Asthma attacks Symptom days |
|
Noise |
Myocardial infarction Angina pectoris Hypertension Sleep disturbance |
|
Accident risk |
Risk of injuries from traffic and workplace accidents |
Building material |
SO2 Acid deposition |
Ageing of galvanized steel, limestone, mortar, sandstone, paint, rendering, and zinc for utilitarian buildings |
|
Combustion particles |
Soiling of buildings |
Crops |
NOx, SO2 |
Yield change for wheat, barley, rye, oats, potato, sugar beet |
|
O3 |
Yield change for wheat, barley, rye, oats, potato, rice, tobacco, sunflower seed |
|
Acid deposition |
Increased need for liming |
public funding for education, or reduce support for antismoking programs, it is important for them to understand how their constituents view the value of these services compared with each other as well as with other uses of funds. The goal is simply to understand the trade-offs people are willing to make to get more of a good (or avoid doing without). This is a very useful concept for public policy decisions.
To monetize the impacts of externalities, the committee followed standard practice and defined the monetized value of an externality to an individual as the maximum amount that an individual would be willing to pay to obtain the good. This concept is called willingness to pay, often abbreviated WTP.7
There are both practical and conceptual arguments for attaching monetary values to impacts. One practical reason for monetization derives from the multiplicity of external effects and the difficulty of evaluating them in the context of national policy making. From just a partial list of metrics from external effects of coal-fired power plants, there are deaths from coal transportation, injuries from coal transportation, and water temperature increases from cooling-water discharges. The goal is to make sure that these
effects are factored into decisions related to producing electricity from coal. This requires a method for weighing these metrics against each other and, for policy making, against the costs of reducing the effects.
One class of methods is to use a numeraire to develop weights for each effect and to aggregate them. Indeed, in this report, the numeraire that we use is dollars, with the weights developed through the literature on WTP for reducing a given type of effect. Another class of methods is not to establish specific weights but to use multiattribute utility functions or, more generally, multiple criteria approaches (see, for example, Keeney and Raiffa [1993] and Cohon [2004]). Such an approach has been used in the past in the evaluation of federal water projects, but that approach dealt with three or four metrics at a time, not the dozens of metrics associated with the externalities of energy production and use. In contrast, using a monetary metric to the extent practicable, converting all the metrics into a single unit of dollars, and adding them up produces a single grand metric of all the external effects. This is a powerful result of great potential use to policy makers.
By estimating WTP, a monetary value can be placed on each external effect and then added up, thus producing a single dollar value for total external effects, which represents an estimate of the value that society places on those effects.
In this study, the committee did not have the time or resources to undertake new valuation studies and has therefore relied on existing studies of the monetary values of the externalities that we studied. One important example of a value taken from the literature is commonly referred to as the “value of a statistical life” (or VSL), which characterizes the rate at which people are willing to trade increased risk of death for other goods and services. By observing in many occupational and other settings how much people have been willing to pay to reduce the risk of death (or are paid in compensation for taking additional death risks) or by conducting surveys that ask people how much they are willing to pay to lower their death risks, estimates have been made for the VSL that are used in regulatory decision making around the world, including various agencies in the U.S. government. We used these values in our study, as explained in Chapters 2 through 5.
Using WTP as a monetary metric can make some people uncomfortable. There are some effects that are especially difficult to value (for example, ecological impacts) because there are many components of ecosystem services that people do not understand and for which people retain inherent cultural values. So at first blush, potential ecological impacts from climate change, such as lost polar bear habitat, seem to defy monetization. In practice, estimating the effect on polar bear habitat is very difficult because of the poorly understood relationship between changing greenhouse gas con-
centrations, climate effects, and, therefore, reduced habitat, but the fundamental economic valuation question remains conceptually straightforward: How much of other goods and services (such as education, housing, and health care) would people be willing to give up in exchange for preserving polar bear habitat? The conceptual answer will differ depending on whether there are many polar bears left in the wild or whether there are only a few. Whether a monetary value can be estimated that is accurate enough for use in policy decisions remains a challenge for many ecological services. The committee has used WTP to monetize external effects wherever possible, recognizing its limitations and controversies. Some effects are not monetized at all, and others are monetized with great uncertainty. Indeed, some effects cannot even be estimated, much less quantified, even though we know they exist.
The committee is especially aware that ecological impacts, including impacts on ecosystem services, have not been monetized in this report. Evaluating these impacts economically has a long and challenging history (for example, EPASAB 2009; NRC 2004a; Cropper 2000). Ecological effects that influence the production of economic goods, such as agricultural products, timber, fish, and recreational benefits, often have been monetized, although often incompletely. This report includes some aspects of agricultural production in its monetization of the damages from emissions from electricity generation that contribute to the formation of criteria air pollutants. However, changes in ecosystem services, such as nutrient cycling and provision of habitat, and more subtle changes in ecosystem functioning that can affect ecosystem performance have not generally been monetized, largely because it is difficult to quantify those changes at present (for example, Cropper 2000). Although the committee has described these impacts qualitatively, at least to some degree, they likely are significant monetarily and otherwise.
Despite these limits, the commmittee believes that using its results will improve federal policy making.
Consideration of External Benefits
There are obviously considerable benefits to having energy. Most of these benefits are reflected in the prices paid for energy and are not external benefits. For the most part, external benefits are relatively few in number and small compared with the external damages that have been identified. For example, ORNL/RFF (1992-1998) identified the crop fertilization benefits of the nitrogen and sulfur from NOx and SO2, respectively; the crop fertilization benefits of CO2; and the recreational benefits of enhanced fishing opportunities in reservoirs formed from large hydro projects.
Of those, our study explicitly considered the crop fertilization benefits
of CO2; the results of the integrated assessment models considered in Chapter 5 account for the impacts. However, we did not explicitly consider other external benefits for the following reasons: ORNL/RFF (1992-1998) found the crop fertilization benefits of NOx and SO2 to be small compared with the health-effect damages. We did not consider reservoir recreational benefits because we did not consider hydropower as important, for the purposes of this study, as the other technologies considered (refer to Chapter 2).
THE POLICY CONTEXT FOR THIS STUDY
Externalities are important to analyze and understand because they provide an example of a situation where government involvement can potentially improve on the market outcome. Although the committee was not tasked to make recommendations for policy makers to address energy-related externalities, we did indicate how knowledge about the value of externalities can be used to improve market outcomes. This section relates the results of our study to existing policies that address externalities and discusses how the results of the study should and should not be used.
The Nature of Externalities Evaluated in This Study
As noted earlier in this chapter, the committee evaluates the externalities associated with energy production and consumption that have not been corrected through existing policies—that is, the externalities remaining after policies have been implemented. Therefore, the study does not document the substantial progress that has been made in reducing the external damages associated with energy production and consumption over the past few decades. To illustrate, emissions from electric power plants that contribute to criteria pollutant formation are regulated by a variety of state and federal regulations. In particular, one of the goals of Title IV of the 1990 Clean Air Act Amendments was to reduce SO2 emissions from coal-fired power plants by 50% from 1985 levels by the year 2010. Most of the reductions were already achieved by 2005, the year of this study. We quantify the damages associated with remaining SO2 emissions from fossil-fueled power plants in 2005. A similar statement can be made regarding tailpipe emissions from motor vehicles. Emissions from cars per mile traveled have declined by 90% since the passage of the 1970 Clean Air Act as a result of various regulations. We calculate the remaining emissions from cars in 2005 and 2030.
We evaluate the damages associated with emissions in the years of 2005 and 2030, relative to zero emissions. For example, in the case of coal-fired power plants, we characterize the per plant aggregate damages associated with SO2 emissions in 2005 compared with no SO2 emissions. The same is true of the air-pollution damages associated with motor vehicles: We
evaluate the per vehicle total damages from current emissions relative to zero emissions. This is not because emissions should be reduced to zero but because any other baseline would be arbitrary. The appropriate level of SO2 emissions from power plants depends on the costs of reducing those emissions (see Figure 1-1), but estimating the appropriate level of emissions is beyond the scope of this study. The methods used to estimate air-pollution damages from fossil-fueled power plants and motor vehicles assume that the damages of each additional ton of pollution from a source are constant8—hence, we also compute the damages per ton of pollutant, which could be compared with control costs.
In the case of power plants, we provide estimates of the distribution of air-pollution damages across power plants. This is important for two reasons: First, the damages associated with a plant depend on where the plant is located, so damages vary spatially; second, total damages vary greatly across plants because of differences in plant size and pollution control. Variation in damages across plants is useful information from the perspective of pollution control. Plants with large total damages may warrant further air-pollution controls.
We also distinguish damages by the stage of the life cycle at which they are generated. Although it is possible to aggregate NOx damages associated with passenger transportation across all stages of the life cycle—oil exploration and extraction, oil refining, transportation of gasoline to the consumer, and consumption of gasoline by a car—regulations to limit NOx emissions will be targeted at different stages of the life cycle: Regulations to limit tailpipe emissions will differ from those to limit oil-refinery emissions. Similarly for damages associated with electricity generation, it is important for policy purposes to separate mining damages from those damages associated with electricity generation because policies to control each set of externalities will differ. Thus, although we present aggregate estimates of damages—per kilowatt hour or per mile traveled—they should be placed into proper context for policy.
Policies to Correct Externalities
Policies to address or correct externalities include taxes, transferrable pollution permits, performance standards, and technology-based standards. Economic theory dictates that the most efficient policies for correcting
externalities are those targeted at the externality itself—for example, a tax on SO2 emissions rather than a tax on the electricity associated with those emissions or a tax on NOx emissions from motor vehicles rather than a tax on gasoline.9 Taxing SO2 emissions (or regulating them through a permit market or performance standard) provides an incentive to reduce SO2 by using pollution-control equipment, by switching to low-sulfur coal, or by reducing the level of electricity produced. A tax on electricity generation does not provide the incentives to reduce SO2 emissions per se. The same is true of a gasoline tax and NOx emissions.
For emissions related to criteria pollutants, the committee therefore notes that its estimates of externalities associated with emissions per kilowatt-hour of electricity produced or per gallon of gasoline should not be interpreted as recommendations for electricity or gasoline taxes equal to these monetized damages. Economically efficient methods of correcting emissions that contribute to criteria air pollutants include taxes on the emissions themselves or permit markets in which rights to pollute are denominated in terms of damages.10 A similar statement can be made for CO2 emissions. For fossil-fueled power plants, we provide estimates of the damages per ton for key emissions that contribute to criteria air pollutants, as a function of plant location. For CO2 emissions, we provide ranges of estimates of marginal damages.
Externalities and Technology Choice
A frequent use of estimates of the externalities associated with electric power generation and transportation is to inform technology choices when making public investment decisions. Should expansion of electricity-generating capacity take the form of coal, natural gas, nuclear power, or wind power? What technologies should be pursued as alternatives to gasoline-powered internal combustion engines for passenger vehicles? This study can help to inform such choices; however, it must be emphasized that we evaluate the externalities associated with various technologies independent of their costs. For example, an integrated gasification-combined cycle (IGCC) coal plant with carbon capture and storage is an extremely clean plant, but it is also an expensive one. Externalities are an important com-
ponent of the choice among various technologies but must be supplemented by estimates of private costs.
SOME METHODOLOGICAL ISSUES: SPACE, TIME, AND UNCERTAINTY
Defining and evaluating externalities is unavoidably complicated by their spatial nature, by the fact that they manifest themselves over time, perhaps very far into the future, and by uncertainty. We discuss each of these issues in this section.
Spatial Scales of Analysis
The external effects of energy, by their very nature, vary spatially. Some individuals and groups experience far greater effects from energy production and use than is reflected by the average amount—that is, than if the effects were evenly distributed—and others experience far less. In carrying out the committee’s task, we focused on the spatial distribution of damages caused by coal-fired and gas-fired power plants wherever they were located and by transportation emissions in each of the U.S. counties in the 48 contiguous states. Note, however, that a lack of location data for stages upstream of the power plants and vehicle operations prevented us from estimating these kinds of damages in a spatially explicit manner.
Consideration of Effects on U.S. vs. Global Scales
Although the committee’s task stipulated that the external costs and benefits of energy be analyzed from a U.S. perspective, we were also charged with consideration of broader, more global, implications when warranted and feasible. Some effects considered by the committee occur mainly in the United Sates, such as effects related to ozone-forming emissions from motor vehicles. However, other effects, such as those related to CO2 emissions and climate change, will occur on a global scale. Likewise, for some of the security-related issues, or for transportation, which relies on energy production and distribution occurring outside the United States, ignoring the global consequences would result in substantial distortions. Moreover, as is apparent for climate change-related effects, some parts of the world are likely to suffer inherently different, and to some extent larger, burdens of these effects than the United States. In such situations, we have elected to characterize effects both in the United States and on a global scale, as consideration of them on different spatial scales might have an impact on policy choice. For practical reasons, we have provided sparing detail regarding differential impacts among non-U.S. regions.
Consideration of Differential Effects on Local and Regional Scales Within the United States
Within different locations in the United States, many external costs and benefits related to energy are heterogeneously distributed as well—for reasons inherent to the nature of the economic activity or the geography or as a consequence of one or another policy choice. For example, one of the substantive health consequences of climate change in the United States is the impact on heat- and cold-related morbidity and mortality, for example, heat waves. These impacts are far stronger in northern cities with moderate climates within which temperatures fluctuate widely year to year. Because of differences in the extent of human physiologic adaptation to higher temperatures, more people die in heat waves in Chicago than, say, in Birmingham, Alabama, and rising average temperatures will accentuate that disparity further. Likewise, because of greater population density and prevailing winds, the distribution of harmful effects from emissions that form criteria air pollutants is highly nonhomogeneous. For example, populations in eastern seaboard counties bear more of the health-related external costs of this external impact of electricity production from fossil fuels than do populations in upwind areas and will continue to irrespective of any short-term policy choices. Thus, when aggregate damages are presented, the differential impact may be partly obscured.
For other impacts, such as the local—potentially devastating—effects of a power-plant disaster or disruption occurring in a distribution line (for example, an oil or gas line), local choices may be extremely important in determining “who pays.” Often, siting of these types of facilities is partially determined by geographical factors, such as where production and utilization actually occur.
In some situations, aggregate damages may be juxtaposed against local damages, creating not only heterogeneity but also complex policy alternatives. For example, there is at least some evidence that centralized, rather than decentralized, management of spent nuclear fuel results in an inherently lower risk of adverse external consequences; yet arguably for the site or sites chosen for a centralized activity the local “costs” can be higher. Another similar consideration is that the damages of power-plant emissions vary by the population affected by the emissions.
Differences in Susceptibility over Spatial Scales
Even within the same locations, there is compelling evidence that some parts of the human population or that some species within an ecosystem are more vulnerable than others to a particular external effect. One of the factors responsible for differential effects is age; the very young and the very
old are more susceptible to energy-related burdens, such as those imposed by heat stress, water constraints, or pollution. Likewise, the underlying health status of individuals or groups creates large disparities in effects. In highly developed societies, risks of the nutritional consequences of climate change or diarrheal illness are essentially nil, while these impacts dominate in societies with lower levels of overall health status. Conversely, air pollutants from electricity or transportation tend to affect, to a greater degree, individuals and societies with higher underlying rates of cardiovascular and chronic disease, which are more prevalent in richer societies. This same factor differentiates the consequence to an individual with chronic disease from his/her healthy partner, even living in the same house. These conditions may be confounded by disparities created by differential access to resources, for example, socioeconomic differences, within a nation or region. For example, during the last highly publicized heat wave—Chicago 2003—almost all of the excess deaths occurred among poor minorities without air-conditioning or ready access to health or social services. Once again, aggregate cost data would tend to mask, rather than emphasize, such differences.
Temporal Issues
Some effects related to the production and use of energy may take years, decades, or longer to manifest themselves. For example, chronic health effects of air pollution attributable to fuel combustion are not the consequence of an exposure that occurred yesterday or a few weeks ago, but they are the cumulative result of conditions that develop over longer periods. As a more extreme example, health risks from the disposal of nuclear waste generated from electricity production may persist over millennia because of the long-lived nature of the radioactive waste. This persistence presents challenges in making judgments about the performance of a waste repository, the behavior of human society, and other key factors over a very long period.
One challenge is that it is very difficult to predict both the future physical effects and their monetary values because they depend on a host of uncertainties about how people in the future will live. A second challenge arises in comparing effects that are quantified in monetary values at different times (such as expenditures on control equipment now and fewer adverse health effects in the future). In making such evaluations, two factors should be considered. One is that many opportunities exist for investing resources now to yield future benefits. The future benefits of a proposed action should be compared with the future benefits that could be achieved by investing the same resources in other ways. The other factor is that the
people affected may differ, especially if the delays are long enough that they are necessarily members of different generations.
It is conventional and appropriate to discount future values by a factor that depends on the distance into the future and the discount rate. (It is also necessary to account for future inflation, usually accomplished by valuing all consequences in “real”—that is, “constant” or “inflation-adjusted”—dollars.) In addressing the difficulties that arise in identifying the appropriate discount rate, two approaches are often used. The first is commonly referred to as “descriptive”; the second, as “prescriptive.” The descriptive approach uses a discount rate that is similar to market interest rates, which are market prices that are determined by the interactions of individuals, firms, and other institutions seeking to borrow or save for various time periods. The prescriptive approach is often used for time periods of more than about 30 years, for which market interest rates rarely exist. This approach explicitly considers two factors: the rate at which future generations’ utility should be valued relative to the current generation’s utility (an ethical question), and the rate at which incremental resources will enhance the future generations’ utility (a descriptive question) (see Chapter 5 for further discussion).
Estimates of the appropriate discount rate derived from the prescriptive approach are typically smaller than those derived from the descriptive approach. This divergence raises a number of ethical questions, such as whether individuals and governments currently are consuming too much and investing too little and how much individuals should sacrifice now to potentially benefit many future generations.
For valuation of climate-change effects (see Chapter 5), the discounted value referred to as the social cost of carbon is often used. It is the present-day value of the combined damages and benefits that will occur over many future years if an additional ton of greenhouse gas is emitted today. Estimating the discounted cost involves consideration of current greenhouse gas emissions’ effects on climate over the next century or more, environmental and human welfare effects caused by climate change, how the effects may vary globally, the course of future economic development, the range and likelihood of economic and social effects arising from climate change, and the extent to which human society might adapt to climate change. Because the choice of a discount rate for such long periods involves great uncertainty, the committee does not recommend a particular discount rate for assessing the value of these effects.
Model Selection and Evaluation
The committee made extensive use of computational models to evaluate available knowledge, compare alternative technologies, and provide a
framework to assess damage. The committee recognizes that all models face inherent uncertainties because human and natural systems are more complex and heterogeneous than can be captured in a model. Moreover, the committee also recognizes that once a model is selected and applied, large uncertainties remain regarding input selection and choices of scale. In its selection and use of models, the committee relied on a report of the NRC’s Committee on Regulatory Environmental Models (NRC 2007a), which recommended that models cannot be validated (declared true) but instead should be evaluated with regard to their suitability as tools to address a specific question. In following this approach, the committee first identified its specific questions, then identified the tools available, and finally made model selections.
The committee recognized that its analysis involved five key activities: (1) characterizing a range of technologies that provide electricity, transportation, and heating; (2) identifying the pollutant emissions (and other environmental hazards) attributable to each technology; (3) linking emissions (hazards) to exposures; (4) linking exposures to effects; and (5) translating effects into damages that can be monetized. Modeling was required for electricity production and heating—steps 3, 4, and 5—and for transportation impacts—steps 2, 3, 4, and 5. Therefore, the committee reviewed a number of models that could support this task and considered several alternatives, including several models to address the issues of model uncertainty. Ultimately, the committee determined that the use of a single model would make its results more transparent and open to evaluation than would trying to interpret results from several models. The committee selected the APEEP (Air Pollution Emission Experiments and Policy) model (see Chapter 2) for steps 3, 4, and 5 and the GREET (Greenhouse Gases, Regulated Emissions, and Energy Use in Transportation) model (see Chapter 3) for step 2 in transportation technologies. In making these choices, the committee did not consider these two models to be the only or even the best models for this task. Instead, the choice reflects the committee’s recognition that these models were clearly appropriate for the task, were accessible to the committee, were transparent in their applications, and had received sufficient prior use and performance evaluation. To further evaluate the performance of these models for use in calculating external impacts, the committee carried out comparative evaluations where that was feasible.
Intake Fraction and Other Tools for Model Evaluation
The committee sought other studies with comparable results to evaluate the consistency of its model approach with approaches used by others engaged in similar research. In making these evaluations, the concept of “intake fraction” was useful and transparent. It is defined by Bennett et
al. (2002) as the integrated incremental intake of a pollutant summed over all exposed individuals and occurring over a given exposure time, released from a specified source or source class, per unit of pollutant emitted. Since that time, numerous studies have estimated intake fractions for various source categories (such as power plants, mobile sources, residential wood burning, indoor cleaning products, and aircraft) and pollutants (such as particulate matter and toxic air pollutants). Most important, use of the intake fraction approach has increasingly become a tool for model performance evaluation and model comparisons.
For source-receptor estimates from power plants, work by Nishioka et al. (2002) provided a model evaluation opportunity. To assess the health effects of increased pollution, Nishioka et al. (2002) modeled state-by-state exposures to fine particulate matter (PM2.5) originating from power-plant combustion and used intake fraction as an intermediate output. The committee was able to compare its power-plant intake fraction obtained from APEEP with theirs and got consistent results. Moreover, Nishioka et al. (2002) multiplied their population-weighted exposures derived from intake fractions by exposure-response functions for premature mortality and selected morbidity outcomes, providing the committee with further opportunity to evaluate APEEP results.
In the transportation impact modeling, there were two studies that provide key evaluation opportunities. In an effort to better characterize the relationship between mobile-source emissions and subsequent PM2.5 exposure, Greco et al. (2007) characterized PM2.5 exposure magnitude and geographic distribution using the intake fraction. They modeled total U.S. population exposure to emissions of primary PM2.5 as well as particle precursors SO2 and NOx from each of 3,080 counties in the United States. Their mean PM2.5 intake fraction was 1.6 per million with a range of 0.12 to 25 per million compared with 1.0 per million with a range of 0.04 to 33 per million obtained from APEEP. Greco et al. (2007) concluded that long-range dispersion models with coarse geographic resolution are appropriate for risk assessments of secondary PM2.5 or primary PM2.5 emitted from mobile sources in rural areas but that more-resolved dispersion models are warranted for primary PM2.5 in urban areas because of the substantial contribution of near-source populations. One of the advantages of APEEP is better spatial resolution in urban counties, but it may still lack the necessary level of spatial detail, giving rise to some uncertainty about results.
Marshall et al. (2005) used three alternative methods to estimate intake fractions for vehicle emissions in U.S. urban areas. Their best estimate of the urban intake fraction for diesel particles was 4 per million, results that are consistent with the urban-county results in APEEP. However, the need for future efforts to provide exposure resolution below the county scale remains a priority.
Addressing Uncertainty
Assessment of uncertainty in model outputs is central to the proper use of model results for decision making. There are a number of uncertainties that arise in the calculation of damages from energy use. The committee elected to confront uncertainty using approaches recommended by the NRC’s Committee on Regulatory Environmental Models (NRC 2007a). This committee considered the use of probabilistic (Monte Carlo) approaches to quantify all uncertainties to be problematic in many situations, especially when uncertainty analysis is used to reduce large-scale analyses of complex environmental and human health effects to a single probability distribution or when uncertainty is dominated by decision variables, as is the case for this current study. In this study, uncertainty is dominated by such factors as the selected value of a statistical life, which cannot easily be captured in a probability distribution. In situations where detailed probabilistic modeling is not appropriate, the models committee (NRC 2007a) recommended the use of scenario assessment and sensitivity analysis. The current committee chose to use this approach, and where feasible, it has used alternative scenarios and sensitivity analysis to characterize uncertainties.
ORGANIZATION OF THE REPORT
The discussion in Chapter 2 focuses on the external effects and their valuations, resulting from electricity generation. Chapter 3 addresses externalities related to the production and use of transportation fuels. Chapter 4 discusses energy used to supply heat for industrial processes and to heat indoor spaces. Chapter 5 addresses effects attributable to climate change and their valuations. Chapter 6 discusses effects and valuations related to infrastructure and security. Chapter 7 presents overall conclusions from the committee’s evaluations, including a comparison of climate and nonclimate damage estimates, and discusses factors to keep in mind when interpreting the results of the evaluations. Chapter 7 also recommends research to inform future consideration of various issues in this report.