Biodeterioration of Stone
Thomas D. Perry IV, Christopher J. McNamara, and Ralph Mitchell
Division of Engineering and Applied Sciences
Laboratory of Applied Microbiology
Harvard University
Cambridge, Massachusetts
ABSTRACT
Stone cultural heritage materials are at risk of biodeterioration caused by diverse populations of microorganisms living in biofilms. The microbial metabolites of these biofilms are responsible for the deterioration of the underlying substratum and may lead to physical weakening and discoloration of stone. Air pollutants in urban environments accelerate biodeterioration by serving as an additional nutrient source for the microorganisms. Current strategies to reduce biodeterioration and repair damage that has already occurred are discussed. Current techniques for assessing microbial populations and their effects are evaluated. Additionally, we describe two new techniques for quantification of these interactions: microcomputer-assisted tomography (microCT) and atomic force microscopy (AFM). The study of biodeterioration of stone cultural heritage materials is as diverse as the sites studied. This review also attempts to address some of the issues facing conservation scientists, including methodology and application.
INTRODUCTION
Many cultural heritage materials are at risk of biodeterioration by microorganisms, including metals (e.g., Berk et al., 2001), glass (e.g., Schabereiter-Gurtner et al., 2001), ceramics (e.g., Sand and Bock, 1991), paper (e.g., Fabbri et al., 1997), paintings (e.g., Rubio and Bolivar, 1997), wood (e.g., Bjordal et al., 1999), coatings (e.g., Flemming, 1998), synthetic polymers (e.g., Gu et al., 1998a), and
mummified bodies (e.g., Arya et al., 2001). Biodeterioration presents conservation challenges that vary as widely as the types of materials themselves, and discussion of the processes causing degradation of these varied historic materials is not possible here. Consequently, the scope of this paper is limited to discussion of the mechanisms, analytical techniques, and conservation strategies of stone biodeterioration.
Biodeterioration plays an important role in the degradation of stone in historic buildings, monuments, and archaeological sites (e.g., Saiz-Jimenez, 1999). Microorganisms that have been demonstrated as the causative agents in deterioration of stone include bacteria (Urzi et al., 1991), Archaea (Rölleke et al., 1998), cyanobacteria, algae (Tomaselli et al., 2000), fungi (Gorbushina et al., 1993), and lichens (Garcia-Rowe and Saiz-Jimenez, 1991). Additionally, stone objects may support novel communities of microorganisms (e.g., alkaliphiles, halophiles, and endoliths) that function in the biodeterioration process (Saiz-Jimenez and Laiz, 2000). Our work focuses on Maya archaeological ruins in the Yucatán, Mexico, which are heavily colonized by microorganisms (see Figure 1). We have isolated copiotrophic (capable of growth on a medium containing a high concentration of organic carbon), alkaliphilic (capable of growing at high pH), oligotrophic (capable of growth on media containing a low concentration of organic carbon), and halophilic (salt-tolerant) bacteria, as well as phototrophic microorganisms and

FIGURE 1 Photograph of a room in the Quadrangle of the Nuns, Uxmal, Yucatán, Mexico, showing visible microbial growth and staining.

FIGURE 2 Scanning electron micrograph (30,000X) of an unidentified microorganism with an unusual morphology collected from the surface of an inner room in a temple in the Maya site at Uxmal, Yucatán, Mexico.
fungi from the ruins. Unique organisms that have to date eluded our identification efforts have also been observed (see Figure 2).
Microbial biodeterioration of stone occurs as a result of the formation of biofilms (see Figure 3). Biofilms are collections of bacterial cells on surfaces that are maintained by electrostatic forces and/or adhering exopolymers. Biofilm formation begins with the initial adhesion of microorganisms to a surface. Division of attached cells produces microcolonies containing large amounts of exopolymer separated by patchy areas relatively devoid of growth. Production of exopolymer and other exudates is stimulated in response to cellular density by cell-cell signaling. The exopolymer matrix is composed mainly of polysaccharides and serves a variety of functions such as providing protection from desiccation, radiation, erosion, and disinfectants, as well as storage of organic carbon and nutrients (Flemming and Wingender, 2001; Costerton et al., 1995). The exopolymer matrix limits the rate of diffusion in microcolonies, resulting in the formation of microenvironments due to gradients in pH, O2, nutrients, and organic carbon (Rittman et al., 1999).
EFFECTS OF MICROBIAL METABOLITES
Effects of Acids
Microbial biodeterioration of stone is widely thought to occur through the action of organic and inorganic acids produced as metabolic by-products (Gu et
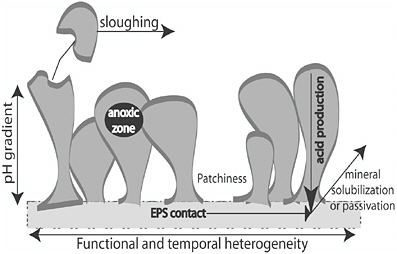
FIGURE 3 Diagram of a microbial biofilm growing on a stone surface, which highlights the environmental heterogeneity present in attached microbial communities.
al., 2000). Bacteria isolated from the Maya site of Ek’ Balam, Yucatán, Mexico, are capable of producing calcium carbonate-dissolving exudates (Perry et al., 2003). However, not all organic acids produced by microorganisms cause immediate dissolution of stone. For example, oxalic acid may have a protective role by the formation of calcium oxalate on stone surfaces (Di Bonaventura et al., 1999).
Effects of Exopolymers
In addition to metabolic acids, biofilm exopolymers may play an important role in deterioration of stone because of their proximity to the stone substratum. Bacteria produce the polymers as biofilm growth is initiated, and adhere directly to the stone (see Figure 4). Bacterial exopolymers are large macromolecules consisting of varied sugar molecules exhibiting several kinds of functional groups (Ford et al., 1991), including acidic carbonyls. These functional groups are often capable of binding cations in solution (Smidsrød and Haug, 1965). For example, negatively charged carboxylic and hydroxyl groups of exopolymeric materials, such as alginic acid, form complexes with the mineral surface and may leach calcium from limestone matrices (Perry et al., 2004). Other polysaccharides with different chemistry may inhibit dissolution (Welch and Vandevivere, 1994). The activity of these molecules and their functional groups in chelation and dissolution of stone is still not understood because of their variability and complexity.

FIGURE 4 Scanning electron micrograph (30,000X) showing bacteria growing on a marble surface and producing exopolymer attached to the stone substratum.
Discoloration
Microbial pigments frequently cause discoloration of stone. While these metabolites may not cause physical damage, they can cause aesthetic problems. Monuments are particularly susceptible to this form of discoloration.
A typical example is the red stain observed on the U.S. Naval Academy’s Tripoli Monument in Annapolis, Maryland (see Figure 5a). Two pigment-producing fungi were isolated from the monument: Epicoccum nigrum and Drechslera sp. Interestingly, in a growth medium of low salt concentration, E. nigrum appeared milky white in color, while in a high calcium concentration medium, the fungus produced a red pigment (see Figure 5b). The red stain may have been an exudate produced to protect the fungus from the stresses of its habitat on the stone, such as ultraviolet radiation or ionic strength (Wheeler and Bell, 1988).
INTERACTION OF MICROORGANISMS WITH AIR POLLUTANTS
One of the most serious causes of stone deterioration is urban air pollution caused
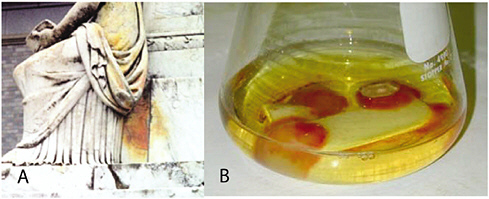
FIGURE 5 (a) Red pigmentation on the Tripoli Monument in Annapolis, Maryland. (b) Production of pigment by the fungus Epicoccum nigrum in a growth medium containing marble. The fungus was colorless when grown on the same medium without addition of marble.
by fossil fuel combustion. In addition to chemical weathering of stone, pollutants may stimulate microbial biodeterioration. Urban air pollutants are rich in both nitrogen dioxide (NO2) and sulfur dioxide (SO2). NO2 and SO2 are mainly derived from fossil fuel combustion and are transported by wind or water to stone surfaces (Saiz-Jimenez, 1993). Some chemoautotrophic bacteria obtain energy by oxidizing sulfur and nitrogen compounds to sulfuric and nitric acids. For example, Thiobacillus colonizes weathered surfaces of marble in a polluted area (Mitchell and Gu, 2000). These bacteria, through production of sulfuric acid, cause degradation of acid-sensitive materials, including limestones and concrete (Gu et al., 2000; Gugliandolo and Maugeri, 1990; Sand, 1994). The reaction of stone carbonate with sulfuric acid also causes the formation of gypsum. The contribution of microorganisms to gypsum formation is unknown. Gypsum crystals combine with dust, aerosols, and other atmospheric particles to form black or brown sulfated crusts, which can tarnish the monument’s aesthetic appearance. The composition of these crusts varies and is dependent on the particular airborne pollutants in individual areas (Saiz-Jimenez, 1993). Furthermore, effects of gypsum formation are not limited to aesthetic problems. While gypsum may temporarily passivate the limestone, the crust may ultimately exfoliate, causing extensive deterioration (Gauri and Holdren, 1981).
Nitrifying chemoautotrophic bacteria are able to derive energy by oxidizing nitrogen-containing inorganic substrates (Mansch and Bock, 1996), ultimately resulting in the formation of nitric acid. The effect of biogenic nitric acid attack on stone was investigated and was compared to the effects of a smoggy atmosphere (Mansch and Bock, 1996). Mansch and Bock (1996) indicated that micro-
biologically formed nitric acid corrosion was eight times more harmful than the corrosion caused by smog.
The relative significance of chemical and biological processes in most locations is unknown. It is probable that there is synergism between the two processes. There is extensive evidence that in urban environments, a wide range of hydrocarbons is adsorbed to historic buildings. The growth of hydrocarbon-utilizing bacteria on these buildings may increase the deterioration rate of the stone (Mitchell and Gu, 2000).
ANALYSIS OF MICROBIAL POPULATIONS AND PROCESSES
Microbial growth on stone has traditionally been analyzed using methods that rely on the ability of microorganisms to grow on culture media. In recent years, however, molecular techniques that exploit natural variation in DNA sequences have been developed to enumerate and identify microorganisms. Culture-based and molecular methods each have advantages and disadvantages. For example, despite the simplicity and ease of use of culture-based techniques, they routinely detect less than 1 percent of microorganisms present in environmental samples (Pace, 1997). Very often the physiological state observed in culture does not represent the organism’s activity in situ (Bonin et al., 2001). Molecular techniques avoid the selective bias of culturing but require significant expertise and may introduce other sources of error especially in natural samples containing multiple species’ templates (Thompson et al., 2002). The inability to detect the majority of organisms limits the usefulness of culture-based techniques for studies in which the goal is an accurate description of the microbial community. However, in studies where the goal is a comparison between treatments, analysis of a fraction of the community may be sufficient (Lemke et al., 1997). Furthermore, molecular analyses yield little information about the function of organisms. Molecular methods are insufficient for investigating the production of metabolic products and for investigating the effects of these products on mineral dissolution. These data are required if we are to understand the underlying processes of deterioration.
In addition to phylogenetic descriptions, the deterioration of stone has been examined using a variety of techniques, which have some utility in the quantification of biodeterioration. These methods include depth measurements using calipers, use of reference surfaces, macro-stereophotogrammetry, ion measurements of water runoff, or acid extraction (Winkler, 1986). Scanning electron microscopy (SEM), which is used to observe surface degradation, fails to detect changes below the surface. Nuclear magnetic resonance (NMR) measures changes in pore size distribution within stone (Alesiani et al., 2000) but gives no information about the microorganisms. Both SEM and NMR require destruction of the sample. Acoustic wave velocity provides information about subsurface discontinuities in
stone (Papida et al., 2000). However, results generally must be correlated with other measurements, such as change in stone mass.
X-ray computed tomography (CT) has been used extensively for the nondestructive visualization of objects in medical research (Berland, 1987), paleontology (Conroy and Vannier, 1984), soil, and sediments (Phogat and Aylmore, 1989). Recently attempts have been made to adapt CT for use with stone samples from building materials (Jacobs et al., 1995). These standard CT machines are large and have poor resolution. A recently developed small desktop micro computed X-ray tomography (MicroCT) was used to analyze stone samples at high resolution (Ruegsegger et al., 1996). In addition to being nondestructive, MicroCT provided images of the interior and exterior of stone objects, as well as three-dimensional reconstructions of the samples. Marble blocks were exposed to 1 mM sulfuric acid, which is typically associated with acid rain, and the effect was assessed by MicroCT according to McNamara et al. (2002). Exposure to 1mM sulfuric acid caused little change in mass, surface area, or solution pH value. An 18 percent increase in surface area was observed using MicroCT, possibly indicating the formation of a gypsum crust protecting the stone from further dissolution. A three-dimensional reconstruction of horizontal scans can be seen in Figure 6. MicroCT is a useful technique for quantifying the effects of microorganisms and their exudates. MicroCT is also of use for long-term studies, because it is nondestructive and allows for repeated scans on the same sample. Quantitative density measurements can be recorded over time.
Atomic force microscopy (AFM) has previously been used to study the kinetics of calcite dissolution when exposed to water and simple acids (e.g., Shiraki et al., 2000). Using a time series of AFM micrographs (see Figure 7), a geometric analysis of surface topographical changes can be used to calculate a microscopic dissolution rate. The AFM chamber can be concurrently used as a flow-through reactor to determine macroscopic dissolution rates (Shiraki et al., 2000) with
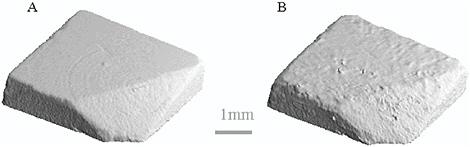
FIGURE 6 A three-dimensional reconstruction of microCT planar scans of a marble sample before (a) and after (b) treatment for four days with 1 mM sulfuric acid. The surface shows formation of a gypsum layer.

FIGURE 7 An atomic force micrograph of a pit forming in a calcite surface. By monitoring the step velocity of the pit expansion a dissolution rate can be calculated.
methods described in Duckworth and Martin (2003). The AFM may prove useful for quantifying the effect of bacterially produced acids and polymers on stone dissolution (Perry et al., 2004).
STRATEGIES FOR CONTROL OF BIODETERIORATION
Environmental Control
Microorganisms can persist in dry environments. Active metabolism, however, requires appropriate levels of relative humidity and temperature. A combination of low humidity and low temperature is the simplest way to control microbial growth, but this treatment may be less effective for control of fungi (Gu et al., 1998b) and is impractical in outdoor situations. Regular cleaning may be the most effective treatment for preventing biofilm formation and subsequent biodeterioration of materials in historic buildings and monuments (Krumbein et al., 1992).
Biocides
The application of biocides has become a routine practice in the conservation of cultural heritage materials. However, environmental issues have severely limited the number of available effective biocidal chemicals for use in conservation (Bingaman and Willingham, 1994). Biofilm bacteria respond differently to biocides and are generally more resistant than unattached cells (McFeters et al., 1995). Because microorganisms are capable of rapidly acquiring chemical resistance, no one chemical can be relied on for long-term use; frequently several chemicals need to be combined in order to achieve effective eradication of biofilm populations. Biocides are a difficult tool for preservation, because many are too caustic for environmental use, they are not strong enough to discourage microbial growth, or the microorganisms ultimately develop resistance.
Consolidants
Consolidants have been used for some time to conserve archaeological stone from biological and chemical weathering (Selwitz, 1992). Consolidation is a means of generating structural strength in disintegrating material and is an artificial means of repairing the damage caused by natural processes (Crafts Council, 1992). The efficacy of consolidants on outdoor stone is controversial, because they can upset the natural saturation and evaporation of moisture from within the stone, often resulting in exfoliation and cracking of stone surfaces (Boyes, 1997). Application of consolidants is not easily reversible, which is a serious drawback when dealing with ancient monuments. Some consolidants may also discolor as they degrade because of aging, photochemical processes, and oxidation (Biscontin et al., 1976; Gonzalez, 2000).
Two of the most common types of consolidants used for monuments and archaeological stone are ethoxysilanes and acrylic resins. Commercial consolidants are susceptible to biodegradation (Koestler et al., 1994). A wide range of consolidants has been tested for effectiveness to protect Maya limestone in Belize (Kumar and Ginell, 1995), and most of the polymers tested proved to be susceptible to microbial degradation under local environmental conditions of the Yucatán. However, guidelines are needed for systematic evaluation of candidate polymers and their suitability in specific applications. Physical conditions for biodeterioration may be particularly favorable in tropical and subtropical regions because of high temperatures and humidity.
While problems associated with the use of consolidants for the protection of archaeological stone are numerous, they are only one of the means of preventing the disintegration of stone grains due to exposure and weathering. The addition of biocides to consolidants would help to prevent microbial degradation, increasing the longevity of the treatment. Commercially available and environmentally acceptable biocides could be used as additives in consolidants.
CONCLUSIONS
Stone cultural heritage materials are constantly at risk of deterioration by microorganisms. This risk is augmented in urban environments, where deposition of pollutants enhances the rate of deterioration. Microbiologists are actively pursuing the microorganisms responsible for deterioration and attempting to quantify their effects. The effects of metabolic products, the deterioration processes, and the responsible microorganisms are all being elucidated. This effort, however, requires a multidisciplinary examination of the geology, chemistry, and conservation of these sites.
ACKNOWLEDGEMENTS
The authors would like to thank M. Breuker (National Park Service, Lowell, Mass.), M. Muilenberg (School of Public Health, Harvard University, Boston, Mass.), R. Muller (Beth Israel Deaconess Medical Center, Boston, Mass.), and J. Sembrat (Conservation Solutions, District Heights, Maryland.) for their collaboration on aspects of this manuscript. This work was supported in part by a grant from the National Science Foundation (BES-9906337). T. D. Perry is supported by a Samuel H. Kress Fellowhip.
REFERENCES
Alesiani, M., S. Capuani, F. Curzi, and B. Maraviglia. 2000. In International Congress on Deterioration and Conservation of Stone, ed. V. Fassina, pp. 579-585. Venice, Italy: Elsevier.
Arya, A., A. R. Shah, and S. Sadasivan. 2001. Current Science 81:793-799.
Berk, S. G., R. Mitchell, R. J. Bobbie, J. S. Nickels, and D. C. White. 2001. International Biodeterioration and Biodegradation 48:167-175.
Berland, L. L. 1987. Practical CT: Technology and Techniques. New York: Raven Press.
Bingaman, W. W., and G. L. Willingham. 1994. International Biodeterioration and Biodegradation 34:387-399.
Biscontin, G., S. Frascati, and L. Marchesini. 1976. In The Conservation of Stone, pp. 731-747. Bologna: Centro per la Conservazione delle Sculture all’Aperto.
Bjordal, C. G., T. Nilsson, and G. Daniel. 1999. International Biodeterioration and Biodegradation 43:63-71.
Bonin, P., J.-F. Rontani, and L. Bordenave. 2001. FEMS Microbiology Letters 194:111-119.
Boyes, N. 1997. In Aspects of Stone Weathering, Decay, and Conservation: Stone Weathering and Atmospheric Pollution Network Conference (SWAPNET), eds. M. S. Jones and R. Wakefield, pp. 170-178. London: Imperial College Press.
Conroy, G. C., and M. W. Vannier. 1984. Science 226:456-458.
Costerton, J. W., Z. Lewandowski, D. E. Caldwell, D. R. Korber, and H. M. Lappinscott. 1995. Annual Review of Microbiology 49:711-745.
Crafts Council. 1992. In Adhesives and Coatings, pp. 123-131. London: Conservation Unit of the Museums and Galleries Commission in conjunction with Routledge.
Di Bonaventura, M. P., M. D. Gallo, P. Cacchio, C. Ercole, and A. Lepidi. 1999. Geomicrobiology Journal 16:55-64.
Duckworth, O. W., and S. T. Martin. 2003. Geochimica et Cosmochimica Acta 67:1787-1801.
Fabbri, A. A., A. Ricelli, S. Brasini, and C. Fanelli. 1997. International Biodeterioration and Biodegradation 39:61-65.
Flemming, H. C. 1998. Polymer Degradation and Stability 59:309-315.
Flemming, H. C., and J. Wingender. 2001. Water Science and Technology 43:1-8.
Ford, T., J. Black, T. Kelley, R. Goodacre, R. C. W. Berkeley, and R. Mitchell. 1991. Applied Environmental Microbiology 57:1595-1601.
Garcia-Rowe, J., and C. Saiz-Jimenez. 1991. International Biodeterioration and Biodegradation 28:151-163.
Gauri, K. L., and G. C. Holdren, Jr. 1981. Environmental Science and Technology 15:386-390.
Gonzalez, R. F. 2000. In International Congress on Deterioration and Conservation of Stone, vol. 2, ed. V. Fassina, pp. 235-243. Venice, Italy: Elsevier.
Gorbushina, A. A., W. E. Krumbein, C. H. Hamman, L. Panina, S. Soukharjevski, and U. Wollenzien. 1993. Geomicrobiology Journal 11:205-221.
Gu, J.-D., T. E. Ford, and R. Mitchell. 2000. In Uhlig’s Corrosion Handbook, 2nd ed., ed. R. W. Revie, pp. 477-491. New York: John Wiley.
Gu, J.-D., D. B. Mitton, T. E. Ford, and R. Mitchell. 1998a. Biodegradation 9:39-45.
Gu, J.-D., M. Roman, T. Eesselman, and R. Mitchell. 1998b. International Biodeterioration and Biodegradation 41:25-33.
Gugliandolo, C., and T. L. Maugeri. 1990. In The Conservation of Monuments in the Mediterranean Basin, ed. F. Zezza, pp. 221-224. Brescia, Italy: Grafo.
Jacobs, P., E. Sevens, and M. Kunnen. 1995. Science of the Total Environment 167:161-170.
Koestler, R. J., E. Santoro, L. Koepp, M. Derrick, J. Druzik, F. Preusser, A. Rodarte, and N. Valentin. 1994. In Research Abstracts of the Scientific Program: GCI Scientific Program Report, ed. J. R. Druzik, pp. 132-133. Marina del Rey, Calif.: Getty Conservation Institute.
Krumbein, W., J. Braams, G. Grote, M. Gross, K. Petersen, V. Schostak, and T. Warscheid. 1992. In The International Conference, ed. R. G. M. Webster, pp. 237-238. Edinburgh: Donhead.
Kumar, R., and W. S. Ginell. 1995. In Methods of Evaluating Products for the Conservation of Porous Building Materials in Monuments, pp. 163-178. Rome, Italy: ICCROM.
Lemke, M. J., B. J. Brown, and L. G. Leff. 1997. Microbial Ecology 34:224-231.
Mansch, R., and E. Bock. 1996. In Microbially Influenced Corrosion of Materials, eds. E. Heitz, H.-C. Flemming, and W. Sand, pp. 167-186. Berlin: Springer.
McFeters, G. A., F. P. Yu, B. H. Pyle, and P. S. Stewart. 1995. Journal of Industrial Microbiology 15:333-338.
McNamara, C. J., T. D. Perry, M. Breuker, and R. Mitchell. 2002. In Microbially Influenced Corrosion, Corrosion—2002, ed. B. Little, pp. 113-121. Houston: NACE International.
Mitchell, R., and J. D. Gu. 2000. International Biodeterioration and Biodegradation 46:299-303.
Pace, N. R. 1997. Science 276:734-740.
Papida, S., W. Murphy, and E. May. 2000. In 9th International Congress on Deterioration and Conservation of Stone, ed. V. Fassina, pp. 609-617. Venice, Italy: Elsevier.
Perry IV, T. D., O. W. Duckworth, C. J. McNamara, S. T. Martin, R. Mitchell. 2004. Environmental Science and Technology 38:3040-3046.
Perry, T. D., C. J. McNamara, G. Hernandez-Duqué, and R. Mitchell. 2003. In Molecular Biology and Cultural Heritage , ed. C. Saiz-Jimenez, pp. 137-140. Lisse: A. A. Balkema.
Phogat, V. K., and L. A. G. Aylmore. 1989. Australian Journal of Soil Research 27:313-323.
Rittman, B. E., M. Pettis, H. W. Reeves, and D. A. Stahl. 1999. Water Science and Technology 39:99-105.
Rölleke, S., A. Witte, G. Wanner, and W. Lubitz. 1998. International Biodeterioration and Biodegradation 41:85-92.
Rubio, R. F., and F. C. Bolivar. 1997. International Biodeterioration and Biodegradation 40:161-169.
Ruegsegger, P., B. Koller, and R. Muller. 1996. Calcified Tissue International 58:24-29.
Saiz-Jimenez, C. 1993. Atmospheric Environment, Part B—Urban Atmosphere 27:77-85.
Saiz-Jimenez, C. 1999. Geomicrobiology Journal 16:27-37.
Saiz-Jimenez, C., and L. Laiz. 2000. International Biodeterioration and Biodegradation 46:319-326.
Sand, W. 1994. Werkstoffe und Korrosion 45:10-16.
Sand, W., and E. Bock. 1991. International Biodeterioration and Biodegradation 27:175-183.
Schabereiter-Gurtner, C., G. Pinar, W. Lubitz, and S. Rolleke. 2001. Journal of Microbiological Methods 47:345-354.
Selwitz, C. M. 1992. In Materials Research Society Symposium, vol. 267, pp. 925-941. Los Angeles: Getty Conservation Institute.
Shiraki, R., P. A. Rock, and W. H. Casey. 2000. Aquatic Geochemistry 6:87-108.
Smidsrød, O., and A. Haug. 1965. Acta Chemica Scandinavica 19:329.
Thompson, J. R., L. A. Marcelino, and M. F. Polz. 2002. Nucleic Acids Research 30:2083-2088.
Tomaselli, L., G. Lamenti, M. Bosco, and P. Tiano. 2000. International Biodeterioration and Biodegradation 46:251-258.
Urzi, C., C. Lisi, G. Criseo, and A. Pernice. 1991. Geomicrobiology Journal 9:81-90.
Welch, S. A., and P. Vandevivere. 1994. Geomicrobiology Journal 12:227-238.
Wheeler, M. H., and A. A. Bell. 1988. In Current Topics in Medical Mycology, vol. 2, ed. M. R. McGinnis, pp. 338-387. New York: Springer-Verlag.
Winkler, E. M. 1986. APT Bulletin 18:65-70.