3
Physiological Interventions
The coral holobiont is the result of a complex metagenome comprising the coral host and its algal symbionts and the other microbiome members including fungi, prokaryotes (bacteria and archaea), and viral components. Reef corals respond to changes in their environment through mechanisms that can originate either in the coral host or in its microbiome. Consequently, reef coral health and function depend on the responses of, and interactions between, all of these partners. Here we describe potential interventions that can influence the physiological responses of reef corals without changing their individual genomes. This includes pre-exposure of the holobiont to environmental stress to elicit compensatory responses that increase their resistance or resilience to future stress, changes in the composition of reef coral metacommunities (in favor of more stress-tolerant algae or other microbes with therapeutic benefits), and the use of antibiotics, phage therapy, antioxidants, and nutritional supplements.
These novel approaches can compensate for the impacts of environmental stress in different ways and at different points in the stress response. For example, oxidative stress caused by high temperatures might be avoided by hosting more thermotolerant algal symbionts or having higher constitutive expression of heat shock proteins. Alternatively, high levels of oxidative stress might instead be managed using beneficial bacteria to detoxify reactive oxygen species as they are produced. Finally, bleached corals might be treated with nutritional supplements to help them recover from bleaching. These different interventions vary in their longevity, with the effects of some triage-based activities being short-lived
(days to weeks), while other activities might be longer-lasting (months to years). Some interventions might have effects that remain for the lifespan of the coral host (typically decades), while others might have impacts that are passed on to future generations.
PRE-EXPOSURE
What It Is
Various terms have been used to describe the processes by which prior exposure of an organism to certain (usually stressful) environmental conditions induces a response that better prepares it for subsequent re-exposure. These terms include “pre-exposure,” “pre-conditioning” (or sometimes just “conditioning”), “induced acclimatization,” “priming,” “stress hardening,” and even just “acclimation.” Additional terms have also been used to refer to the mechanisms by which these responses are achieved (e.g., “constitutive upregulation” or “frontloading” for gene expression) or to refer to the observable change in organisms that results (e.g., “phenotypic plasticity” for physiological or morphological responses). Here, we use the term “pre-exposure” as a general term to refer to the deliberate exposure of an organism (in this case, reef corals and their symbionts) to conditions that might, by whatever mechanism, confer some degree of additional tolerance to subsequent re-exposure of the organism (or in some cases, its progeny) to the same or similar conditions. This term places the emphasis on the initial exposure itself as the intervention being considered, rather than the mechanism by which the increase in tolerance is achieved (whether it be genetic, epigenetic, physiological, behavioral, etc.). The initial exposure can be either acute or chronic, might involve multiple stressors, and could involve either a single shock exposure or incremental increases over time. The induced response prepares the organism for conditions that may also be either acute or chronic. Given this wide variety of exposure-response combinations, it is to be expected that different mechanisms will vary in their importance depending on the timescales involved in both exposure and response.
Benefit and Goals
It has long been recognized that prior exposure of organisms to stressful conditions can shape their response to subsequent re-exposure to the same or similar conditions. In fact, this phenomenon is so fundamental that its existence can be argued from first principles: If organismal responses to stress are designed to help them compensate, then the degree to which these responses prepare the organism for subsequent exposure
to the same stress is simply dependent on how long the organism maintains the response once the initial exposure has ended.
In reef corals, evidence demonstrating that pre-exposure has a beneficial effect (whether or not the specific mechanism is known) is widespread. It is well known that corals in lagoons and on reef flats routinely experience high temperature and high light stress (and sometimes high or low salinities and aerial exposure), resulting in individuals that are tolerant of thermal bleaching events, although the relative contributions of local selection versus pre-exposure can be difficult to determine (Palumbi et al., 2014). That some part of this response is due to pre-exposure was first shown by Brown et al. (2000), who found that reef flat coral surfaces chronically pre-exposed to high solar irradiance by virtue of facing west versus east were more resistant to thermal bleaching, indicating that prior experience of chronic stress could shape the response of corals to acute stress of a different (but related) variety, even when they were the same genotype (see also Brown et al., 2002).
Over larger scales, coral reefs prone to high temperature variability tend to experience less bleaching in response to episodic thermal stress than reefs with more constant temperatures (McClanahan and Maina, 2003; McClanahan et al., 2005, 2007; Safaie et al., 2018). This indicates that certain pre-exposure regimes, such as high temperature variability, can be beneficial in producing corals that are better able to deal with acute stress, although the longevity of the protective effect and the role of selection versus acclimatization remain difficult to determine. Similar conclusions are also implicated in the discovery by Ainsworth et al. (2016) that temperature regimes that pre-expose reefs to mild thermal stress (above the maximum monthly mean temperature, but below the bleaching threshold) for short periods (1-2 weeks) can protect corals from subsequent bleaching a few weeks later, provided the initial pre-exposure is followed by a brief “recovery” period of cooler temperatures. Pre-exposure of corals to high irradiance may also help protect them from bleaching through the production of photoprotective fluorescent proteins, which can reduce oxidative stress on corals (Salih et al., 2000, 2006).
The value of pre-exposure as an intervention strategy to increase the resilience of coral reefs (largely by pre-exposure of corals to high temperature or high light to increase their bleaching tolerance) remains dependent on how long the compensatory response lasts once the initial exposure has ended (or how readily chronic exposure can be maintained). This, in turn, is likely to be dependent on the mechanisms involved. Four types of mechanisms can be broadly distinguished: (1) acclimatory changes in gene expression, (2) adaptive changes in gene expression, (3) epigenetic modification, and (4) changes in holobiont composition. These phenomena are described in Box 3.1 and in the Algal Symbiont Manipulation section.
Although some of these mechanisms, such as acclimatory changes in gene expression, can result in compensatory changes that can be almost instantaneous and highly reversible (e.g., the upregulation of genes expressed in basic metabolism or cellular function in response to higher temperature), other changes can take much longer to develop and can potentially last much longer. These include changes in gene expression that result in structural changes to gross morphology or cellular architecture (especially in early ontogeny) and adaptive changes in gene expression that can be permanent due to DNA alterations (eQTLs). Other long-lived changes include epigenetic modifications (that can sometimes be heritable), and changes in symbiont diversity or dominance. All of these changes can apply to different members of the coral microbiome, not just the coral host itself. For example, physiological changes in algal symbionts exposed to high light include changes in the abundance of symbionts, the amount of chlorophyll per cell, the size of photosynthetic units, and xanthophyll cycling capacity (Brown et al., 2002), all of which can influence the subsequent bleaching tolerance of the coral host, while also differing in how long these responses last.
How to Do It
Pre-exposure interventions can be divided into three general categories that vary in their approach and differ in the degree to which they can be effectively implemented or scaled. The first approach (and most ambitious from the point of view of scalability) is acute pre-exposure. This involves the short-term exposure (typically days to weeks) of corals (at
the larvae, recruit, or adult colony stage) to certain conditions at a specific time in order to trigger a response with long-lasting effects. The timing of the exposure may be key. Examples include exposing adult corals to high temperatures during gametogenesis or larval development, rearing larvae or settling recruits under stressful conditions prior to their release to the reef, or bleaching corals during early spring and allowing them to recover on the reef during the warming summer months. The second approach is chronic pre-exposure, which involves the long-term (months to years) exposure of (typically adult) corals to a particular set of conditions with the expectation that corals will trigger a variety of mechanisms which might together result in tougher corals. An example is the establishment of coral nurseries in relatively stressful (e.g., inshore) environments designed to effectively anticipate future conditions experienced by corals once outplanted.
The third approach is to assume that natural environmental variation already experienced by corals in the wild results in individuals that have already benefited from pre-exposure, and to exploit the benefits of this long-term (years) pre-exposure when identifying corals for restoration. An example is sourcing corals from local environments that have already been exposed to stressful conditions and prioritizing these for grow-out in a nursery. This latter approach shares some aspects with managed selection, but focuses on the benefits of site-specific acclimatization, although the difference may be hard to recognize without genetic testing.
Current Feasibility
Pre-exposure is currently feasible in the laboratory or in small-scale restoration efforts. Acute exposure in the laboratory is technically feasible at small scales, requiring informed decisions regarding life stage, duration, and degree of stress. Establishing coral nurseries in naturally stressed environments is more easily implemented because it is essentially a matter of identifying an appropriate nursery location. Identifying corals that have been naturally “pre-exposed” to stress is less straightforward to implement because it requires some knowledge of coral distribution and environmental variation, but it is nevertheless still very feasible. Studies described in Box 3.1 show that coral react to stress on a cellular level, with varying degrees of longevity of the response.
Potential Scale
The different pre-exposure approaches vary in their scalability. This reflects the fact that, although the science behind these responses is well understood in most cases, no prescriptive strategies for pre-exposure
have been developed or implemented. Methods of acute pre-exposure, such as stress-hardening corals in nurseries prior to outplanting, may be straightforward to test and implement on an individual basis, but hard to scale. This may be especially true for adult colonies because each individual must be managed and monitored to ensure appropriate dose. Pre-exposure in batches as part of a restoration outplanting program will help these activities to be scaled up, but it will still require significant expenditure of time and resources. Acute pre-exposure of gravid corals to particular conditions (in the expectation that their gametes or larvae will be more tolerant of these conditions) may be more scalable because pre-exposure of one colony may translate to hundreds or thousands of pre-exposed gametes or larvae. Similarly, pre-exposing larvae or recruits may be scalable, but only if an active larval rearing and seeding program is already in place.
Chronic pre-exposure of adult corals in the nursery phase as part of an outplanting program may be more readily scalable, because it only involves identifying an appropriate location for the nursery. Similarly, provenancing strategies that exploit existing environmental conditions to naturally pre-expose colonies to appropriate conditions may also be very scalable, and are similar to managed relocations, which are considered in Chapter 4. The pre-exposure value of managed relocations would be in addition to the value of any fixed (genetic) effect due to local selection at the source site.
Beyond the spatial constraints of scaling, the value of pre-exposure is likely to be limited by the effectiveness and longevity of the compensatory response. Certain types of responses (such as acclimatory and reversible changes in gene expression) might only last minutes to hours, while other types of responses (such as changes in symbionts and epigenetic modifications) might potentially last for the rest of the organism’s lifespan, or even be passed on to the next generation (see Figure 3.1). Consequently, it is difficult to generalize.
Risks
The principal risk of pre-exposure is that prior exposure to stressful conditions actually weakens the organism concerned, rather than strengthening it. This might result due to the expenditure of energetic resources used in activating the desired response and might be exacerbated by “overdoing” it—applying excessive amounts of stress in the pursuit of the beneficial response. The degree to which these risks are likely depends on the approach used and its anticipated mechanism. For some approaches, the likelihood of hormesis—where a beneficial response is induced following the application of a low dose of a stressor
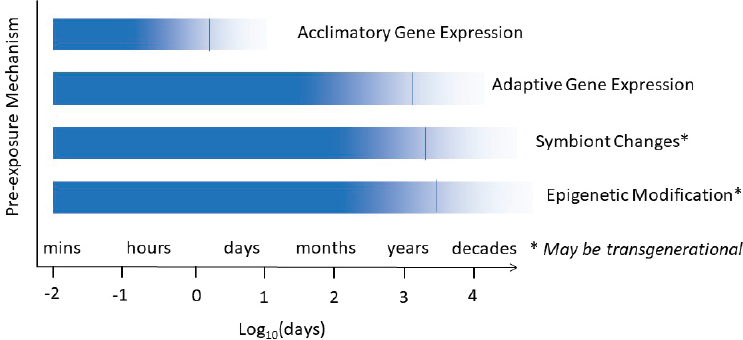
that would be harmful at higher doses—may be more likely than others. However, the majority of these approaches have not been attempted in the field and consequently the risk of “doing more harm than good” still needs to be tested.
Limitations
Pre-exposure approaches are largely limited by the ability to scale up in space and time. In almost all cases, longevity is likely to be influenced by the environmental conditions that the organism experiences following the initial period of pre-exposure. Conditions that are similar to those to which the organism was pre-exposed are more likely to extend the duration over which the compensatory mechanism remains in operation.
Infrastructure
Infrastructure needs depend on the life stage at which these preexposures might be implemented. Acute pre-exposure at the larval or recruit stage requires an active gamete/larvae collection and rearing program and an effective recruit seeding program. In contrast, chronic pre-exposure or managed relocation of adult colonies would require an effective nursery propagation and outplanting program.
ALGAL SYMBIONT MANIPULATION
What It Is
The algal symbionts of scleractinian corals (hard corals) have long been known to be diverse (Baker, 2003; Blank and Trench, 1985; Rowan and Powers, 1991). A recent revision of these symbionts recognized a new dinoflagellate family, the Symbiodiniaceae (LaJeunesse et al., 2018), comprising numerous genera corresponding to what were formerly clades of the single genus Symbiodinium (a genus that is retained to refer to certain members of clade A). To date, members of many of these genera (corresponding to former Symbiodium clades A, B, C, D, F, G, and H) have been detected in scleractinian corals (Quigley et al., 2018a), with multiple symbiont types occurring among different colonies of the same coral species (Rowan and Knowlton, 1995) and within colonies (Rowan et al., 1997).
Different symbiont types have been shown to influence the stress phenotype of the host, particularly in response to high temperature (Baker et al., 2004; Glynn et al., 2001) or high light (Rowan et al., 1997) disturbance, and these are often reflected in the distribution of these symbionts over environmental gradients (Baker et al., 2004; Fabricius et al., 2004; Iglesias-Prieto et al., 2004; Rowan and Knowlton, 1995). These differences are most striking when the symbionts belong to different genera within the Symbiodiniaceae. However, differences in stress phenotype, both within genera (e.g., Cladocopium, formerly Symbiodinium clade C) and within species (Parkinson and Baums, 2014), also exist, indicating that functional diversity exists at many taxonomic levels (Suggett et al., 2017). It is now clear that corals of the same species hosting different symbionts typically vary in their physiological capabilities, and corals with stress-tolerant symbionts typically have higher bleaching thresholds. Thus, there have been attempts to manipulate algal symbiont associations to favor partnerships that confer stress tolerance to coral. The degree to which corals can change their symbiont communities in favor of these stress-tolerant partnerships, the coral life stage at which it is most appropriate to attempt these changes, and the consequences of doing so remain topics of active research.
Another approach that has been used to modify algal symbiont communities is to use artificial selection in the laboratory to experimentally produce symbiont lineages that are more thermotolerant (Chakravarti and van Oppen, 2018; Chakravarti et al., 2017). These symbionts can then be used for experimental manipulations involving corals, principally at the recruit stage, but also potentially in adults. Although conceptually similar in practice to many of the procedures outlined below, some of the risks associated with the use of this form of “experimental evolution” are more similar to those outlined in Assisted Gene Flow and Assisted Migration in
Chapter 4, including certain genetic risks and tradeoffs, such as selection for one trait (e.g., high thermotolerance) coming at the expense of other traits.
Benefit and Goals
Changes in symbiont communities, at the individual colony level, can result in changes to holobiont phenotype, potentially helping corals respond to changes in the environment, such as higher irradiance (Baker, 2001; Rowan et al., 1997) or warmer temperatures (Baker et al., 2004; Berkelmans and van Oppen, 2006; LaJeunesse et al., 2009). For example, bleached corals have been observed to recover with symbiont communities dominated by Durusdinium (formerly Symbiodinium clade D; LaJeunesse et al., 2018). This genus contains several thermotolerant species (e.g., D. trenchii, D. glynnii) that confer higher bleaching resistance to their coral hosts (Berkelmans and van Oppen, 2006; Cunning et al., 2015b, 2018; Glynn et al., 2001; Grottoli et al., 2014; Jones et al., 2008; LaJeunesse et al., 2009; McGinley et al., 2012; Rowan, 2004; Silverstein et al., 2015, 2017). Indeed, shuffling to Durusdinium can increase bleaching thresholds by approximately 1°C-2°C (Berkelmans and van Oppen, 2006; Silverstein et al., 2015). Corals with D. trenchii also appear to retain their symbionts during periods of cold stress, even though the symbionts are impaired, suggesting that some Durusdinium may also be resistant to expulsion in general (Silverstein et al., 2017). High- and low-temperature stress tolerance may be of benefit to corals in particular areas, for example Florida, where corals have suffered recent mortality as a result of both high- and low-temperature stress (Lirman et al., 2011).
How to Do It
Adult corals Although changes in algal symbiont communities have been observed in the field, typically in response to coral bleaching (Baker et al., 2004; LaJeunesse et al., 2009) or transplantation (Baker, 2001; Berkelmans and van Oppen, 2006; Rowan et al., 1997), deliberate manipulation of symbionts in adult corals has to date only been achieved by duplicating these disturbances in the laboratory through controlled bleaching and recovery. These manipulations have exploited the fact that, while coral bleaching often leads to mortality when environmental stress (e.g., high temperature) is prolonged and/or severe, corals can recover their symbionts within a period of weeks to months if the stress is alleviated (Glynn, 1993). Moreover, the loss and subsequent re-establishment of Symbiodiniaceae communities through bleaching and recovery can present an opportunity for these communities to change (Baker, 2001;
Buddemeier and Fautin, 1993), either through changes in the relative abundance of different symbiont types, or acquisition of different symbionts from the environment (symbiont “shuffling” versus “switching”; Baker, 2003). To date, three species of Caribbean coral (Montastraea cavernosa, Siderastrea siderea, and Orbicella faveolata) have been manipulated in the laboratory, by exposure to moderate heat stress (32°C for 10 days), followed by recovery under cooler conditions. This “stress-hardening” treatment is a form of pre-exposure and is therefore conceptually similar to the pre-exposure interventions discussed earlier. Controlled bleaching and recovery boosted the abundance of D. trenchii from near 0% to over 99%, while causing almost no mortality in experimental corals (Cunning et al., 2015b, 2018; Silverstein et al., 2015; see Figure 3.2). Moreover, the degree of symbiont community change could be reliably predicted based on bleaching severity and the photochemical advantage of one symbiont over another in the coral species in question. Methods for applying this approach to nursery-reared corals prior to outplanting as part of a restoration program are currently undergoing trials in Florida, and involve the

application of acute bleaching stress and/or the rearing of corals under chronic nonbleaching stress as means of modifying symbiont communities (Andrew Baker, personal communication).
Coral recruits The majority of scleractinian coral species (approximately 85%), including most broadcast spawners, produce gametes that do not contain algal symbionts. These species must by necessity acquire Symbiodiniaceae from environmental sources (horizontal transmission). A minority of species (approximately 15%) contain symbionts in their eggs, and these symbionts are considered maternally supplied (vertical transmission). Few species exhibit both strategies (Babcock and Heyward, 1986; Baird et al., 2009b; Fadlallah, 1983; Harrison and Wallace, 1990). Consequently, for the majority of species, algal symbionts must be obtained de novo each generation, at the larval or early recruit stage, and this represents a clear opportunity for intervention. This is particularly true because the specificity of coral species for particular Symbiodiniaceae appears to be much lower in early life history stages than it is in the adult, although there is considerable variation among corals species and these systems still remain far from “open” (Poland and Coffroth, 2017; Quigley et al., 2016, 2017). Coral larvae or juveniles typically acquire diverse symbiont assemblages which are then “winnowed” during early ontogeny to a dominant symbiont (or, occasionally, subset of symbionts) that is more typical of the adult, which may itself be dependent on the prevailing environmental conditions (Abrego et al., 2009; Coffroth et al., 2001, 2006; Dunn and Weis, 2009).
The natural predisposition of most, if not all, coral larvae or juveniles to acquire algal symbionts from environmental sources (LaJeunesse et al., 2004, 2010a) suggests they could be intentionally infected with algal symbionts that have phenotypes of interest, such as high thermotolerance. This could be achieved using either lab- or field-raised recruits (Quigley et al., 2018b). Such symbionts could be naturally occurring (Little et al., 2004), selected for in the laboratory (Chakravarti and van Oppen, 2018; Chakravarti et al., 2017), or genetically manipulated (see Genetic Manipulation in Chapter 2). In fact, early life history stages are likely the most effective way of effecting changes in symbiont communities, because these stages are predisposed to symbiont acquisition and are already aposymbiotic (symbiont-free), which means that existing communities of symbionts need not be displaced or outcompeted in order for others to become dominant, as is the case with adult corals. In fact, the window during which symbionts may be readily acquired may persist for several months (Abrego et al., 2009; Boulotte et al., 2016; McIlroy and Coffroth, 2017) and likely continues into adulthood (Coffroth et al., 2010; Lewis and Coffroth, 2004). Indeed, it seems likely that symbiont acquisition from
environmental sources occurs throughout a coral’s life cycle, but is simply difficult to detect or conclusively demonstrate due to the large population sizes of symbionts in adult corals, and because it is almost impossible to determine with certainty whether novel symbionts are acquired from environmental sources or were simply present at low abundance somewhere in the colony beforehand.
Directed laboratory selection Chakravarti et al. (2017) artificially selected for higher thermotolerance in C. goreaui (formerly Symbiodinium C1) symbionts over 80 generations (2.5 years) using laboratory incubators. They employed a ratchet design, in which temperatures were increased in stepwise increments, with cells showing growth at each temperature being used to seed cultures at the next higher temperature. The evolved symbionts were then used to infect Acropora recruits and assess their effects on growth and bleaching severity under thermal stress. These methods were generally able to select for symbionts with improved photochemical performance and higher growth rates when cultured at elevated temperatures (31°C), but the increased thermotolerance acquired when free-living was less apparent in symbiosis with the coral host. In a follow-up experiment, Chakravarti and van Oppen (2018) showed that similar methods could be used to select for thermal tolerance in a variety of different genera within the Symbiodiniaceae (former Symbiodinium types A3c, F1, and G3) over the course of approximately 1 year (41-69 generations) but were not successful in increasing the thermotolerance of (normally already thermotolerant) Durusdinium. Future work is likely to focus on whether artificial selection for positive cell growth rates necessarily translates to higher bleaching resistance, and on the longevity of the increased thermotolerance once the selective pressure is removed. Regardless, the practical considerations of manipulating corals using experimentally evolved symbionts, particularly during the early life history stages, are similar whether the symbionts are naturally occurring or experimentally evolved.
Current Feasibility
Adult corals For adult corals, the principal feasibility concerns are related to effective scaling. Laboratory bleaching is feasible, but unlikely to be practical given the need to manipulate large numbers of corals. Symbiont manipulations might be included as part of restoration efforts involving the asexual propagation of adult corals in nurseries, because significant time is already being invested in fragmenting, propagating, and outplanting individual fragments. Treatments designed to modify algal symbiont communities might be incorporated into the design or placement
of nurseries and/or growout practices. For example, corals could be bleached in the field by exposing them in the short-term to high doses of solar irradiance or high-temperature stress just prior to outplanting (e.g., extremely shallow depths or reef-flat environments), and in fact such practices are currently being trialed in both the laboratory and the field in Florida (Andrew Baker, personal communication). Alternatively, nurseries could be established in locations where the environmental conditions might be expected to favor particular symbionts even in the absence of bleaching. The success of these methods in changing symbiont communities will likely depend on the coral species used, and on the environmental characteristics of the nurseries and/or outplant sites. Even if successful in changing symbionts, these approaches will still require an extra step in the restoration process, and their large-scale adoption by restoration practitioners will be more likely if the benefits of these manipulated symbiont communities are readily apparent, such as by a significant reduction in bleaching mortality.
Coral recruits Manipulation of the early life stages of corals to introduce particular symbiont types is possible with existing technology and methodology. In fact, the deliberate infection of coral juveniles with particular symbionts, followed by outplanting to natural reef environments, has already occurred on the Great Barrier Reef (albeit as part of a small-scale experiment, rather than a large-scale intervention strategy; Little et al., 2004). The application of these methods may not yet be feasible in areas where successful recruitment is limited by other biotic or abiotic factors, such as Florida and parts of the Caribbean. However, given the interest in developing methods for improving recruitment in these areas, this approach seems poised for potential inclusion into these methods as and when they are implemented.
Potential Scale
Adult corals Symbiont manipulations operate at the scale of the individual coral colony, and are thus subject to significant scaling limitations. However, as colonies containing manipulated symbionts are introduced to reefs, this could increase the local availability of these symbionts on reefs over time. New recruits to these reefs might benefit from the increased availability of thermotolerant symbionts, which may also become more common as temperatures increase and/or bleaching events become more frequent and severe. Similarly, adult colonies already present on the reef might also benefit from higher symbiont availability under these conditions. Consequently, there may be potential positive feedbacks that
operate at the level of the individual reef that could increase the long-term effectiveness of these approaches. However, these feedbacks are largely uninvestigated from a research perspective.
Coral recruits Scaling limitations are slightly alleviated for coral recruits, due to the high numbers of recruits that could be treated with preferential symbionts during an ex situ rearing or grow-out phase. Although this method still operates at the level of the individual, the small size of recruits, and the large numbers that are typically produced during a spawning event mean that such interventions might be scaled up as part of a managed breeding approach.
The long-term fate of these symbionts in corals is likely to depend on the environmental conditions at the restoration site, with warmer conditions naturally favoring thermolerant symbionts, and cooler conditions favoring thermosensitive symbionts (LaJeunesse et al., 2009) that translocate more photosynthate (Cumbo et al., 2018). Consequently, the long-term value of introduced symbionts will depend critically on the future environmental conditions at the site of interest, and on the coral species of interest. Infected corals must maintain at least some of these symbionts over long time scales (probably at least several years), and these symbionts must have value in increasing resilience to the intended stressor, such as thermal bleaching. However, there have been very few studies on the longevity of these associations in the field, where coral recruits were specifically infected with particular symbionts (in the laboratory or other ex-situ facility) and then outplanted to the reef. Little et al. (2004) showed that Acropora recruits experimentally infected with different symbionts maintained these symbionts for at least 6 months on the reef, using symbionts that are commonly found in these corals. In contrast, Coffroth et al. (2010) successfully infected bleached Porites with symbionts that included types that did not normally associate with these corals, but found that infected corals reverted to their typical associations after 5 weeks. Therefore, the risk of symbiont manipulations failing is highly dependent on the identities of both the host and symbiont.
Risks
The manipulation of algal symbiont communities in favor of particular traits (such as higher thermotolerance) is likely to be accompanied by tradeoffs, regardless of how these symbionts are obtained (through isolation and culture, experimental evolution, or transgenic modification). Symbionts that are naturally more thermotolerant, such as some members of Durusdinium, impart greater thermal tolerance to their coral
hosts but grow more slowly (Jones and Berkelmans, 2010; Jones et al., 2008; Little et al., 2004; Pettay et al., 2015), likely because they translocate less photosynthetically fixed carbon (Cantin et al., 2009). This suggests that increases in heat-tolerant symbionts may lead to slower-growing reefs, with implications for the reefs’ ability to maintain coral-dominated states or growth rates that keep up with sea-level rise (Ortiz et al., 2013). However, this tradeoff has also been shown to be temperature-dependent (at least for D. glynnii), with the reduction in coral growth rate associated with D. glynnii decreasing and eventually disappearing at warmer temperatures (Cunning et al., 2015a). Consequently, the benefit of increased survivorship relative to reduced growth is likely to be dependent on the temperature regime at the site of interest. This is also likely true for other tradeoffs associated with the thermotolerance, for example, impacts on host reproductive output and disease susceptibility. However, both positive and negative effects of Durusdinium have been documented on host reproduction (Jones and Berkelmans, 2010; Winter, 2017) and disease susceptibility (Correa et al., 2009), and these different findings likely reflect significant interactions between bleaching and both disease and growth. In bleaching years, corals hosting Durusdinium may bleach less severely and consequently grow faster and have less disease compared to corals that do not host Durusdinium. However, in nonbleaching years these patterns may be reversed. As before, the changing environmental conditions are likely to determine to relative costs and benefits of having different symbionts.
Tradeoffs in experimentally evolved Symbiodiniaceae are also likely to exist, although they have not been conclusively demonstrated to date (Chakravarti and van Oppen, 2018; Chakravarti et al., 2017). Instead, some of the risks associated with the use of experimental evolution are likely to be more similar to those outlined in Managed Selection and Managed Breeding in Chapter 2, in which selection for one trait may come at the expense of others, or local genetic variation is lost. For example, artificial selection for thermotolerance (in this case through mutations accumulated during asexual reproduction of cultures in incubators) might disrupt adaptation to other factors not related to high temperature. Additionally, certain genetic risks of field introductions, such as hybridization with native symbionts as a result of sexual reproduction in the free-living state, leading to outbreeding depression, may be more of a concern for interventions involving experimentally evolved symbionts than they might be for naturally occurring symbionts.
Finally, it has also been suggested that D. trenchii, first described as an “opportunist” by LaJeunesse et al. (2009), might actually be an invasive species from the Indo-Pacific that has spread rapidly through a variety
of Caribbean hosts at numerous sites (Pettay et al., 2015). This points to uncertainty in our understanding of the changing symbiotic milieu on coral reefs (Pettay et al., 2015; Stat and Gates, 2011): Do changes in D. trenchii represent a mechanism that may allow some corals to persist through bleaching events and therefore ultimately prove beneficial given the current climate change crisis (Baker et al., 2004, 2008; Baskett et al., 2009), or are they invasive opportunists whose overall effects, including reductions in coral calcification rate, are deleterious even under climate change scenarios (Ortiz et al., 2013; Pettay et al., 2015)? These questions continue to be areas of active research.
Limitations
In addition to potential tradeoffs, the degree to which symbiont manipulations might be utilized as part of an intervention might be limited by a variety of other factors:
- Specificity and flexibility. At the fundamental level, these interventions depend on the extent to which different symbionts are functionally different from one another (Suggett et al., 2017) and the degree to which different coral species are able to form stable associations with other symbiont types, in particular thermotolerant varieties (Baker, 2003; Cunning et al., 2018). Dynamic changes within individual colonies appear to be common among species that routinely host multiple types (Baker, 2001; Berkelmans and van Oppen, 2006; Cunning et al., 2015b, 2018; Grottoli et al., 2014; LaJeunesse et al., 2009; Rowan et al., 1997; Silverstein et al., 2015, 2017). But it remains unclear whether symbiont manipulation is limited to particular coral species that are unusually flexible. Other species may be far less labile in their associations, severely limiting the utility of this intervention. One way of overcoming this limitation is to apply directed laboratory evolution to symbionts that are already found in the coral host of interest (Chakravarti and van Oppen, 2018; Chakravarti et al., 2017). However, it is not yet clear whether evolved symbionts with higher thermotolerance necessarily result in corals that have greater resistance to coral bleaching.
- Longevity. New symbionts may be lost over time (LaJeunesse et al., 2010b; Thornhill et al., 2006a, 2006b) or experimentally evolved symbionts (Chakravarti and van Oppen, 2018; Chakravarti et al., 2017) may lose their evolved thermotolerance. Both of these processes are likely dependent on the thermal regime at the site of interest.
- Availability. The capacity of corals to undergo changes in symbionts, especially as a result of controlled bleaching and recovery, may be dependent on the local availability of appropriate symbiont; it may be easier to stress-harden corals in some places than others. There is also extreme symbiotic complexity at some sites (e.g., Mexico; Kemp et al., 2014) but not others (e.g., Bahamas; Thornhill et al., 2006b; see also Kennedy et al., 2015, 2017). It is clear that D. trenchii is present throughout the Caribbean in at least some hosts (O. annularis), with varying levels of abundance ranging from trace background levels to virtual dominance (Kennedy et al., 2015). It appears that D. trenchii is increasing in abundance throughout the region, and these increases are likely due to environmental conditions and in particular chronic thermal stress (Kennedy et al., 2017). However, an appropriate long-term study has not yet been undertaken.
Infrastructure
The cost of symbiont manipulations and the infrastructure (personnel, facilities) needed are dependent on how well these activities are integrated into existing coral restoration activities (either asexual or sexual) at the site of interest. If effectively integrated into an asexual restoration program involving coral nurseries and outplanting, costs might be low to implement once a prescriptive bleaching protocol has been developed, or a chronically warm nursery site identified. Costs are likely to be even lower to incorporate symbiont manipulations into a sexual restoration program involving larval rearing and assisted recruitment. In fact, the potential benefits and ease of introducing algal symbionts during coral early life stages are such that failing to incorporate such measures would probably represent a lost opportunity.
MICROBIOME MANIPULATION
What It Is
This section will highlight the current body of knowledge regarding studies undertaken to manipulate the microbiome of corals for building coral resilience. The microbiome in this context is excluding the photosymbiotic dinoflagellates and relatives (manipulation of which is described in the previous section), but includes the fungal, prokaryotic (bacteria and archaea), and viral components of the microbiome. Peixoto et al. (2017) proposed the term Beneficial Microorganisms for Corals (BMCs) to refer to specific microbial symbionts that promote coral
health. The proposed mechanisms by which the BMCs can influence host coral health include facilitation of enhanced nutrient cycling, biological control of potential pathogens, supply of essential trace nutrients, metals, and vitamins plus provision of stress regulators (such as catalase and superoxide dismutase) that mitigate reactive oxygen species (ROS) within the coral holobiont (Bourne et al., 2016; Peixoto et al., 2017). Through augmentation of the beneficial native microorganisms associated with coral, stress response and tolerance mechanisms may be enhanced. However, while the diversity has been assessed extensively (reviewed in Bourne et al., 2016; Huggett and Apprill, 2018), very little is known about the functional attributes of the coral microbiome.
Coral-associated bacterial diversity has been shown to depend on environmental habitat and surrounding environmental conditions (Hong et al., 2009; Littman et al., 2009; Rohwer et al., 2002). Ziegler et al. (2017) recently suggested that coral heat tolerance was causally linked with the associated bacterial community, reporting that corals exposed to different thermal regimes harbored different microbiomes. Importantly, for reciprocally transplanted corals, the microbiomes of corals from stable cooler environments shifted during bleaching conditions while the microbiomes of corals from variable warmer climes, also exposed to bleaching conditions, showed no community shifts, suggesting a role of the microbiome in the response of corals to the heat stress (Ziegler et al., 2017). However, it cannot be determined from this study if the microbiome directly influences host thermal resilience. For other related cnidarian model taxa such as Nematostella vectensis (starlet sea anemone), the fine-scale microbial community composition has been proposed to influence the hosts’ ability to acclimate or even adapt to changing environmental conditions (Mortzfeld et al., 2016).
Microbes occupy a variety of niches within the coral holobiont, and the ability to manipulate them for coral resilience depends on knowledge of their function and composition across these niches.
- Microbial communities in the external nutrient-rich mucus layer are likely influenced by the external environmental conditions surrounding the holobiont, as well as by the exudates from the corals, and are likely to be highly diverse (Bourne and Webster, 2013). In model systems such as Hydra, the bacterial communities residing in this mucus layer are structured and actively cultivated by the host, providing a vital function that supports the host health (Augustin et al., 2017; Deines and Bosch, 2016), although it is unclear if coral can do the same.
- Within the tissues of corals, the microbiome is much less diverse and highly organized within bacteriocytes that have been termed
-
coral-associated microbial aggregates (Work and Aeby, 2014). One common bacterial taxon associated with corals (and a range of other marine invertebrates) is the Endozoicomonas genus (Bayer et al., 2013; Neave et al., 2017). A number of studies that have stressed corals documented a shift in the microbiome, with a reduction in the relative abundance of Endozoicomonas communities associated with healthy corals to microbial communities that are characterized by opportunistic species such as those of the family Rhodobacteraceae (Cardenas et al., 2012; Pollock et al., 2017b; Roder et al., 2014; Sunagawa et al., 2009).
- The skeleton of corals also contains a diverse and distinct microbiome compared to the other niche environments of the coral holobiont (Bourne et al., 2016). This endolithic community can be very different depending on the morphology of the corals (i.e., mounds verses branches) and it is often a mix of eukaryotic algal, fungal, and bacterial communities (Marcelino et al., 2017). Only recently have studies documented the taxonomy and functional attributes of some of these endolithic communities (Yang et al., 2016). It is currently unknown what role if any this microbial endolithic community has in supporting the overlying tissues and the coral holobiont in general. Fine and Loya (2002) looked at bleached corals and demonstrated that during bleaching, the endolithic community bloomed and provided additional nutritional supplementation to the coral tissues.
- The gastric vascular cavity and coelenteron fluid that connects coral polyps through the coenosarc region has been proposed to contain a unique and diverse microbial community that can be influenced by water flow and heterotrophic feeding of the coral polyps (Agostini et al., 2012; Bourne et al., 2016). The bacterial communities in the gastric cavity have been demonstrated to be high in vitamin B12 and hence provide important trace nutrients to the coral holobiont (Agostini et al., 2009).
Benefit and Goals
Microorganisms are central to their host’s physiology, contributing across a range of functions from immune systems, development pathways, and behavior (Bosch and McFall-Ngai, 2011). The microbiome can respond rapidly to the surrounding environment often through rapid adaptive evolution (Elena and Lenski, 2003) and, hence, is suggested to be a powerful influence on coral acclimation and even adaption to rapid anthropogenic driven changes and/or disturbance (Webster and Reusch, 2017). This potential for coral-associated microorganisms to be a driver of
adaptive coral host responses is due to their high abundance, their large diversity (both taxonomically and functionally), and their short generation times (Torda et al., 2017). By shifting the microbiome or changing the relative abundance of functionally important groups, the phenotype of the coral host and subsequently its response to environmental change may be shifted, a process termed microbiome-mediated acclimatization (Webster and Reusch, 2017). The hologenome theory of evolution proposed that microbiome-mediated processes that change host fitness can be under selective pressures (Rosenberg and Zilber-Rosenberg, 2013), although the theory has sparked widespread debate and much controversy (Bordenstein and Theis, 2015; Moran and Sloan, 2015). Many reef invertebrates engage in vertical transmission of their microbial symbionts, facilitating microbiome-mediated transgenerational acclimatization if it also confers lasting adaptive benefits to the coral host (van Oppen et al., 2015b). Hence, through manipulation of the coral microbiome (i.e., changes in abundance and/or addition of new members), the resilience of corals to changing environments may be increased for existing coral populations, including a wide range of native species and genotypes, and potentially passed on to future generations.
How to Do It
There are a number of approaches that can be taken to manipulate the coral microbiome with the aim of improving the resilience of the coral host to environmental stress. Different approaches to manipulate the microbiome of corals may include the following:
- Shifting the abundance of the existing (native) coral microbiome populations through isolation and addition of these native communities to the coral holobiont;
- Addition of new bacterial populations that have functions beneficial to the coral holobiont (which might not be derived from the reef environment);
- Subjecting the coral holobiont to environmental stress to promote selection of microbiome members that may have adaptive traits to confer benefits to the coral host (see Pre-exposure earlier in this chapter); and
- Applying genetic engineering approaches (transposon/CRISPRCas9 systems) to introduce traits in bacteria that are beneficial to coral fitness (i.e., increased ROS scavenging capabilities), and addition of these modified microbial populations into the corals’ normal microbiome (see Genetic Manipulation in Chapter 2).
Examples to date of coral microbiome manipulations and their resulting effects on the coral host are described here:
- Santos et al. (2015) selectively isolated coral-associated bacterial strains capable of degrading water-soluble oil fractions and re-added these strains to corals before exposing them to conditions that simulated an oil spill. Corals exposed to the bacterial additions displayed higher symbiont photosynthetic competence compared to coral treatments without the added bacteria, indicating improved health outcomes.
- Damjanovic et al. (2017) exposed Acropora tenuis larvae to coral-derived microbially laden mucus from different species (Acropora sarmentosa, Acropora tenuis, Diploastrea heliopora, and Galaxea astreata). After subsequent rearing of the larvae for 4 months, the microbial communities associated with A. tenuis juveniles differed across all initial mucus addition treatments. These preliminary studies indicated that just one addition of a mucus/microbial cocktail could shift the coral-associated microbiome during early ontogeny, although no information is available on if or how the microbial communities influenced coral health/fitness.
- Welsh and Vega Thurber (2016) isolated the predatory bacterium Halobacteriovorax from the coral microbiome and showed it could regulate the coral-associated microbial communities through top-down control of certain bacterial species. When added to corals that had also been challenged with the coral pathogen Vibrio coralliilyticus, the predatory bacterium ameliorated changes in the coral microbiome and prevented secondary colonization of opportunistic bacterial groups identified as indicators in compromised coral health (see also Welsh et al., 2016, 2017).
- Rosado et al. (2018) developed a BMC cocktail through isolation of a range of bacterial species that displayed potential beneficial traits for corals including nitrogen fixation and/or denitrification, dimethylsufopropionate degradation, or antagonist activity against putative bacterial pathogens. The BMC cocktail was added to corals in aquariums that were subjected to heat stress to simulate a bleaching event as well as bacterial challenge with the coral pathogen Vibrio coralliilyticus. BMC-inoculated corals displayed improved health compared to noninoculated controls through lower bleaching metrics, demonstrating the potential for addition of BMCs to minimize the impacts of environmental stressors.
Current Feasibility
Approaches to manipulate the coral microbiome are currently feasible at the small experimental scale. Investigated approaches have currently only shown that shifting of the coral host microbiome is possible (Damjanovic et al., 2017) or have demonstrated that addition of microbial isolates is correlated to an improved outcome for the coral host under stress conditions (Santos et al., 2015; Welsh and Vega Thurber, 2016; Ziegler et al., 2017). How the microbiome is shifted at the cellular level and by which mechanisms these benefits are conferred is still currently not understood. The longer-term effects, including whether the shifted microbiome is stable and provides continued benefits to the coral host or if other negative tradeoffs occur is not known.
The approach is also feasible at the level of whole aquarium/grow-out facilities. Like other animal production systems, development of microbial probiotic cocktails that provide benefits to corals is feasible. However, there is much research to be conducted to deduce which microbes are beneficial and at what abundance, and how they can be administered to shift the coral microbiome to increase fitness of the holobiont. These unknowns make it unlikely that benefits will be seen at the reef scales in the near term, but development of improved coral diets that include probiotics, and ways to manipulate the coral microbiome that promote environmental resilience, is potentially achievable with extensive research and development.
Potential Scale
The coral-associated microbiome is generally specific for coral species and even across populations of one species. Hence, approaches aimed at shifting the microbiome are likely required for each specific site and target species (Peixoto et al., 2017). This has applicability for experimental work but also for coral aquariums that may be looking to enhance resilience of corals to be introduced back into the field for restoration.
Inoculation of BMCs could be feasible at reef scales through approaches such as microencapsulation of beneficial microorganisms into “coral food packets” that can be spread onto reefs. Mass production of probiotics exists in both human and animal health fields. However, what is not evaluated or feasible currently is the specific delivery of BMCs into the coral coelenteron. The efficacy of this approach in modulating the coral physiological response to environmental conditions is unknown. Additionally, the delivery systems that could manipulate the microbiome of the target coral species with the desired microbial cocktail specifically, economically, and with minimum risks have not been developed.
On a temporal scale, addition of BMCs could be applied at early and peak periods of environmental stress to minimize physiological impacts, and potentially even following the stress events to help coral recovery. The timing of the addition of microbes to build resilience would be dependent on effectiveness and at what stage in development/growth of corals the microbiome can be modified and if the modified microbiome can be maintained.
Risks
The risks associated with manipulation of the coral microbiome depend on the approach taken. Shifting the relative abundance of naturally occurring (“native”) coral microbiome populations likely represents a relatively small risk as this form of augmentation is minimally invasive and aims to preserve the native microbiome diversity in the reef. However, introduction of high numbers of “foreign” microbes with putative beneficial effects for the coral may drive other unintended processes, including changing pathways of nutrient flow within the coral colony itself or on reefs more generally or introduction of putative pathogens for other reef-based organisms. On the other end of the spectrum, introduction of genetically modified bacterial taxa with engineered benefits for the coral host (such as enhanced ROS scavenging) is associated with many risks requiring full evaluation (see the discussion in Chapter 2 on Genetic Manipulation).
Shifting the microbiome partners may also result in tradeoffs similar to those possible when shifting algal symbionts (i.e., confering thermal resilience but with reduced growth). However, there currently is no information regarding what the tradeoffs may be. Current studies indicate that certain coral-associated bacterial taxa are closely associated with many corals (i.e., Endozoicomonas family); however, under times of stress, these microbial groups decrease in relative abundance in healthy coral and other taxa (i.e., Rhodobacteraceae family) increase in relative abundance (Cardenas et al., 2012; Pollock et al., 2017b; Roder et al., 2014; Sunagawa et al., 2009). This maybe an incidental rather than direct effect of declining coral health, but such taxa can be used as indicators of coral health when looking at tradeoffs (Glasl et al., 2017).
There is also a risk of propagating, incubating, and releasing microbial pathogens into the open reef systems. Disease in any population is always an interaction between the host, the environment, and the causative agent (Wobeser, 2007). In artificial propagation systems, disease is more problematic when there are suboptimal environmental conditions (which can promote pathogen growth and reduce host immune function) and a high host population density (Harvell et al., 2009). Hence, disease
management and quarantine operating procedures need to be optimized to reduce risks of unintended spread of disease into native populations (Sweet et al., 2017). In addition, any added or manipulated members of the coral microbiome would need to be extensively tested to ensure they do not represent disease agents for other coral-reef-inhabiting animals.
Limitations
Currently there is limited knowledge regarding what shapes and maintains the coral microbiome and how the microbiome can be shifted for the benefit of coral fitness, but it is an active field of research. Despite an extensive body of research focused on the diversity of the coral microbiome, few studies have specifically identified the functional attributes individual members of the microbiome provide to the coral host. At present, any microbiome manipulation takes a black box approach. It is assumed that an observed benefit to coral host physiology when adding a microbial cocktail is due to the direct effects of the microbial community. However, the mechanisms at the cellular level that influence the coral hosts’ physiology are unknown and could be due to indirect effects. For example, just adding a microbial cocktail could provide a nutritional component that may enhance the corals physiological resilience. Any mechanistic understanding of the influence of microbiome manipulation on coral physiology would need to resolve which niche the beneficial microbes occupy (i.e., mucus, tissue specific, coelenteron, or skeleton microbial communities). Therefore, understanding the interactions of the members of the coral holobiont at the cellular level is critical to ensure any microbiome manipulation is directly facilitating improved coral fitness and resilience.
These limitations also are influenced by limited knowledge on the most effective approaches for delivering and shifting microorganisms to facilitate higher fitness. For example, increasing the abundance of a known microbial ROS scavenger may have little positive influence if the site of colonization of the microorganism is removed from the sites of ROS production within the coral. It is also unknown whether introduced microbial communities remain associated with corals and influence coral fitness traits over extended periods or into the next generation of corals. There are studies currently focused on transgenerational effects of microbial symbionts on reef invertebrates under future climate scenarios, though the outcomes of these studies are still a work in progress (David Bourne, personal communication). The longer-term effects of shifting the microbiome may not be central if looking at just buffering coral resilience through a single stress event such as a bleaching period; however, if looking to enhance population tolerance over longer time frames, then the question of persistence of the microbiome needs to be considered.
Infrastructure
Infrastructure needs associated with coral microbiome manipulation are principally associated with research and development, specifically in areas of isolation and identification of beneficial microorganisms and testing their efficacy in small-scale aquarium trials. This is due to limited understanding of the specific function of members of the microbiome, how to manipulate them, and if manipulation can be linked to improved outcomes for the coral host. If suitable microbial cocktails are identified and developed, the next steps would be to integrate them into coral nurseries and outplanting sites, taking advantage of the infrastructure established for propagating corals. At these small scales, the costs would not be extensive and application would take advantage of technological applications in other fields, such as agriculture and food production, that currently implement probiotics or microbiome manipulation to boost production. Effective delivery of the microbiome needs to be developed and may take the form of microencapsulation for formulation of coral diets that deliver probiotics/BMCs into corals. These approaches are well developed in other industries and cost effective at limited scale, though, as highlighted previously, the desired specificity and efficacy of these delivery mechanisms does not currently exist. When considering manipulation of the microbiome at large reef scales, there are many unknowns, and infrastructure requirements and costs will no doubt subsequently increase. While industrial production of microbial cocktails is feasible, effective delivery at such large scales has not been currently explored.
ANTIBIOTICS
What It Is
Antibiotics (also known as antibacterials) have found widespread use (and overuse) in the treatment and prevention of bacterial infections in human, animals, and agricultural systems (Allen, 2017; Chang et al., 2015). Antibiotics can be highly effective in the prevention and treatment of bacterial (and some protozoan) diseases. In combination with ease of access to large quantities and cheap cost of production, they can be highly beneficial to many applications spanning human health and large-scale animal production, including valuable marine aquaculture target species (Topp et al., 2017).
Benefit and Goals
Where corals are already displaying signs of stress or disease, therapeutic approaches can improve the health of the individual coral or the
reef ecosystem as a whole. Improvements in corals’ condition through antibiotics (or other therapeutic approaches) might thereafter increase their resilience to environmental stress. In the best-case scenarios therapeutic approaches may even cure that individual or population from impairment in function, for example in a disease outbreak.
Marine diseases are on the rise globally and coral diseases continue to be reported across ocean basins (Burge et al., 2014; Harvell et al., 2004). In the Caribbean, coral disease resulted in mass mortality of many species through the 1980s and 1990s, with these outbreaks decimating the important reef-building branching Acropora species (A. palmata and A. cervicornis) specifically (Aronson and Precht, 2001b), as well as the critically important herbivore Diadema antillarum (Lessios, 2016). A number of bacterial causative agents were implicated in some of the disease outbreaks, although definitive links between the causative agent and the disease lesion is still lacking in many cases (Work et al., 2008). Despite much debate on the causation of diseases (Mera and Bourne, 2018), antibiotic treatment can represent one treatment option to arrest disease spread.
How to Do It
Application of antibiotics could conceivably be used under two scenarios:
- At the localized interface of disease lesions with healthy coral during a field-based disease outbreak. A successful treatment of black-band disease, for example, was implemented using an aspirator device to remove the microbial mat that infected the colony (Hudson, 2000). Subsequently, a modeling clay sealant was pressed into the coral skeleton at the site post-aspiration, reducing reinfection. Inclusion of an antibiotic cocktail into the sealant clay may represent a viable application that improves effectiveness of this dedicated treatment. Experimental trials are lacking in this area and therefore further investigations would be needed to assess applicability, effectiveness, and scalability.
- Many aquaculture facilities historically have integrated broadscale antibiotic treatment into rearing processes when disease outbreaks crippled production (Topp et al., 2017). Applications of antibiotics in coral husbandry and rearing facilities can also be undertaken as a preventative approach to mitigate potential future disease outbreaks. With coral nurseries currently being established at many sites around the world and the growing need for restoration of reef ecosystems, the planned growth of large scale in situ and ex situ coral nurseries is inevitable (Rinkevich,
2005). Within the coral hobby aquarium field, antibiotic treatments are commonly applied and sometime actively promoted across websites and in grey literature publications (Sheridan et al., 2013; Sweet et al., 2011). Often this has involved dosing of the antibiotic into the surrounding water, or removing individual colonies from the tanks and dipping them in an antibiotic bath before placing back into the tanks.
Current Feasibility
Application of antibiotics is feasible currently when applied at small scales either via direct application to individual coral colonies in the field or at the scale of the hobbyist aquariums (Sweet et al., 2011). Larger-scale aquaculture facilities routinely apply antibiotics to high-value marine seafood target species, although the actual figures and efficacy of such approaches can be questioned (Allen, 2017; Watts et al., 2017). Such approaches and operating procedures are similarly applicable to coral aquaculture and propagation facilities.
Potential Scale
Application of antibiotics in situ on coral reefs can be highly contentious, although it is feasible when applied at the scale of treating individual coral colonies. This approach is labor intensive and most efficiently applied in areas that have detailed information on the prevalence of the disease outbreak within the coral population, indicating where individual coral colonies can be targeted to potentially reduce the risk of disease spread. This approach is currently being tried in sections of the Florida Reef Tract where a disease outbreak in recent years has decimated remnant coral colony populations (Erinn Muller, presentation to committee, 2018). In this case, an antibiotic cocktail sealant has been added at the lesion interface to prevent progression of the disease, similar to the approaches tried by Hudson (2000). Application of antibiotics more broadly and diffusely in open coral reef systems including in situ nurseries to enhance resilience of corals to disease outbreaks is feasible, although the effectiveness of such an approach has not been established and is questionable due to dilution effects, the potential for the spread of antibiotic resistance (see Risks section), and other potential unknown impacts.
Application of antibiotics to corals within closed research facilities and larger closed nursery propagation systems is feasible. In experimental systems, the addition of broad-spectrum antibiotics such as ampicillin has been successful in enhancing coral larva settlement and survival, potentially through reduction in antagonistic microbial populations stimulated
via algal exudates or lowering coral resistance to microbial infections (Vermeij et al., 2009). The application of antibiotics is still prevalent in many high-value marine aquaculture facilities globally (Allen, 2017; Topp et al., 2017; Watts et al., 2017) and therefore available dosing protocols, supply, and cost all make antibiotic treatment to prevent disease within closed coral systems feasible on this scale. Most published studies that have used antibiotic treatment on coral, though, have focused on testing causative agents. For example, Sweet et al. (2014) applied ampicillin and paromomycin to Caribbean Acropora cervicornis corals, which arrested the advancing white-band disease type 1 lesions. In studies such as this, treatment is within closed aquarium systems, at very small scale, generally focused on elucidating specific questions around causation and causative agents of disease (Sweet et al., 2014), and not targeted or promoted as therapeutic approaches to improve coral resilience.
Temporal scales also need to be considered when using antibiotics. Often antibiotics have short half-lives and are sensitive to light and temperature. This, along with the rapid dilution of the antibiotics in both closed and open systems, make repeated addition of antibiotics required to arrest disease progression. This contributes to issues such as the rise of antibiotic resistance in target and ancillary microbial populations.
Risks
The inherent risks associated with widespread and rampant use of antibiotics are widely acknowledged in agricultural and human systems (Larsson et al., 2018; Topp et al., 2017). The primary risk is spread of antibiotic resistance through microbial populations that leads current antibiotics to become useless for target organisms (Larsson et al., 2018). Proliferation of antibiotic resistance may occur in the microbiomes of nontarget organisms exposed to the antibiotics as well.
Within the coral context specifically, antibiotic treatments may affect a range of other commensal and potentially beneficial coral-associated microbes, potentially resulting in a destabilized microbiome (dysbiosis) that may subsequently contribute to poor health outcomes for the coral host. Recent studies have highlighted how microbial dysbiosis for coral and other marine organisms is a good indicator of compromised host health (Egan and Gardiner, 2016; Zaneveld et al., 2017). Application of antibiotics, especially as a preventative measure against disease, may therefore destabilize the healthy coral microbiome, making these coral colonies more susceptible to disease. Overall, application of antibiotics and the assessment of risk with coral systems would be needed on a case-by-case basis due to concerns over antibiotic resistance and overuse of antibiotics in environmental settings (Normark and Normark, 2002).
Limitations
There are many limitations related to the use of antibiotics that go beyond the identified risks of spreading antibiotic resistance. For example, in corals, a major limitation is that many causative agents of coral diseases have not been identified (Bourne et al., 2009; Mera and Bourne, 2018). This necessitates the use of broad-spectrum antibiotics, which target bacterial cellular processes that are common for many different bacterial groups, such as inhibition of cell wall structure formation (Kohanski et al., 2010). Use of such broad-spectrum antibiotics therefore results in destabilization of the beneficial microbiome in addition to targeting any coral pathogen implicated in disease. Antibiotic usage in coral systems is also limited by knowledge gaps regarding effective dosages, and delivery mechanisms across different scales, from individual colonies to individual tanks to open reef systems. Additionally, the short half-life due to light sensitivity results in the need for repeated dosing. A major limitation on the effectiveness of antibiotics is that by the time that disease is observed, administration of the antibiotic may be too late to arrest the impacts, making application fruitless (Sweet et al., 2011).
Infrastructure
Antibiotics are readily available and cheap, and a wealth of information on delivery to terrestrial animal, agricultural, and marine aquaculture species has been developed. However, specific application to corals would require the development of technology so that benefits outweigh the risks of antibiotic application and prevent broad release into the environment.
PHAGE THERAPY
What It Is
Phage therapy is the isolation, identification, and application of viruses that specifically target and infect bacteria, known as bacteriophages or simply phages (Abedon et al., 2017). Through infection and lysis of the target bacteria, progression of bacterial-mediated diseases can be arrested (Skurnik and Strauch, 2006). Phage therapy has been a successful approach used in human medicines and was highly advanced especially in Eastern European countries prior to the widespread use of antibiotics (Abedon et al., 2017; Nobrega et al., 2015). Engineered bacteriophages are being developed for human-based trials to counter antibiotic-resistant bacteria that have emerged in the population (Doss et al., 2017).
Benefit and Goals
Today there is renewed interest in using bacteriophages to target a wide range of human disease with bacterial causative agents due to the rise of antibiotic resistance in many previously sensitive bacterial pathogens (Abedon et al., 2017; Torres-Barceló and Hochberg, 2016). There are advantages in the application of phage therapy including the quick and inexpensive preparation of a bacteriophage. In addition, bacteriophages are highly specific to the target bacterial strains making it unlikely that other symbiotic microbes of the coral holobiont are affected (unlike broad-spectrum antibiotics). The bacteriophage population also stays active in the environment that the host inhabits until that host (pathogen) population is below critical levels, meaning fewer or limited applications of the bacteriophage may be required to control the target bacterium (Abedon et al., 2017; Doss et al., 2017; Nobrega et al., 2015; Torres-Barceló and Hochberg, 2016).
How to Do It
The phage therapy approach has been applied to coral in both closed aquariums and open reef environments (Atad et al., 2012; Cohen et al., 2013; Efrony et al., 2007, 2009). The approach takes advantage of the principle that for every bacterium there are one or many bacteriophages that have evolved (or co-evolved) to infect and/or lyse that bacterium (Keen, 2015). Once samples with high viral load have been co-cultured with the target bacterium, any viruses infecting the bacterium will multiply, lyse the bacterium, and then be released into the surrounding medium. Through repeat enrichments, high bacteriophage concentrations can be obtained. For experimental trials, bacteria can be grown on microbial media and the bacteriophage stocks added to see which ones cause clearance of the bacterial lawn. Those that lyse the bacteria can be further purified and concentrated before identification. Once a bacteriophage is isolated and shown to be specific to the target bacterium, it can be grown in large volumes for applications ranging from small to reef scale.
Current Feasibility
Agriculture and aquaculture systems have applied phage therapy to treat plants subject to bacterial-mediated disease and high-value target marine organisms that are often subject to debilitating diseases (Doss et al., 2017). The U.S. Food and Drug Administration has approved application of phage therapy for some crops (Doss et al., 2017). Bacteriophages targeting the putative coral pathogen Vibrio coralliilyticus have been isolated and used effectively to stop advancing white plague-like lesions on
Favia favus coral species in the Red Sea (Atad et al., 2012; Cohen et al., 2013; Efrony et al., 2007, 2009). Hence, development for marine-based coral disease systems is feasible.
Potential Scale
The approach was originally demonstrated to be effective in small-scale experimental systems (Cohen et al., 2013; Efrony et al., 2007, 2009), and has also been shown to be effective in a proof-of-concept study and applied at the reef scale where disease takes hold (Atad et al., 2012). However, the evidence for effectiveness in open-system, reef-scale applications is limited. In theory, because bacteriophages are self-generating entities, one application rather than multiple applications over time may be sufficient. If the target bacterial pathogen proliferates, the bacteriophage will also increase in number (Doss et al., 2017). However, the specific dynamics and practical requirements of temporal applications have not been assessed sufficiently for corals or reefs in general.
Risks
Bacteriophage-mediated lysis of bacterial communities drives much of the cycling of nutrients in oceanic environments (Middelboe and Brussaard, 2017; Roux et al., 2016; Worden et al., 2015), and there is extensive horizontal gene transfer across bacterial/viral lineages in all environments. The application of large numbers of a single bacteriophage to an open reef system does present risks of uncontrolled and unintentional gene transfer events occurring, which may have negative effects on both microbial and macroorganism dynamics. For example, these unintentional effects may include the collapse of bacterioplankton populations that can result in an imbalance of nutrient cycling on the reef. Once bacteriophages are released into an open marine coral reef system, their removal would be extremely challenging or near impossible.
Bacteriophages are self-replicating individuals that have the capability to evolve. Bacteriophage have the potential to spread virulence traits across target and nontarget hosts. Some studies have highlighted that virulence and antibiotic resistance genes exist in some bacteriophage genomes, with the capacity to be passed into hosts that do not have these (Doss et al., 2017; Nobrega et al., 2015). This could result in the unintentional risk of generating new pathogens within an environment. Application of lytic bacteriophages can however minimize the risks associated with incorporation of bacteriophages into host cells and enhanced exchange of genetic information.
Limitations
A limitation to phage therapy is the potential evolution of mechanisms in bacteria to counter bacteriophage infection, allowing emergence of resistance (Doss et al., 2017). Once a bacteriophage is identified and applied, its use is limited and new bacteriophages must be constantly isolated and applied to be effective over longer time scales. While cocktails of bacteriophages that target the same host can partially overcome this obstacle, resistance will eventually arise in the target bacterial population.
Similar to use of antibiotics, another limitation of this approach is the difficulty in conclusively identifying the causative agents of many coral diseases. While bacterial pathogens have been inferred as causative agents of many diseases, there is still debate and a critical lack of understanding of the primary (versus secondary) drivers of disease in corals (Mera and Bourne, 2018). This lack of understanding of the biotic and abiotic drivers of disease will hinder the development of specific bacteriophages to many of the common diseases afflicting corals.
Infrastructure
The costs and infrastructure associated with producing bacteriophage cocktails targeted against coral pathogens is mainly associated within the research and development phase, specifically the isolation and identification of the applicable bacteriophage or bacteriophage cocktails. Once identified and shown to be specific in laboratory-based efficacy trials, the costs and infrastructure required for production and application of the cocktails under small-scale controlled environments are minimal. The technology for delivering a bacteriophage cocktail to corals in open-water reef environments needs development however to establish what is feasible, specific, and effective at this scale.
ANTIOXIDANTS
What It Is
Corals in shallow reef environments reside close to their thermal tolerance and can be exposed to high incident light levels, leading to the production of excess ROS. In corals, the antioxidants superoxide dismutase, ascorbate peroxidase, and cycling of dissolved organic sulfur compounds (DMSP, DMSO, and DMS) likely have big roles in scavenging hydroxyl radicals (Deschaseaux et al., 2014). The addition of antioxidants to corals may provide short-term scavenging (i.e., elimination) of ROS produced during extreme environmental conditions. Similarly, trace metals such as iron play a role in supporting algal symbiont photosynthesis and,
conceivably, their addition may prevent the photosystem breakdown that produces excess ROS (Shick et al., 2011).
Benefit and Goals
During heat-induced thermal bleaching, increased ROS production has been linked to degradation and loss of Symbiodiniaceae cells from the coral host tissues (Lesser, 2006; Smith et al., 2005). Scavenging of ROS is an important cellular process in all animals. However, during environmental stress events such as high seawater temperature periods, particularly in doldrums conditions that promote high light penetration onto reefs, the capacity of the coral holobiont to maintain homeostasis in production and scavenging of ROS becomes disrupted in favor of production (Weis et al., 2008). Heat-tolerant corals potentially enhance ROS detoxification capabilities and this trait is potentially mediated by algal symbiont cells that reorganize their ROS antioxidant network and can be acquired transgenerationally (Levin et al., 2016; Suggett et al., 2017). Enhancing antioxidant activity within the coral holobiont through treatment with ROS scavengers may mitigate environmental stress at the cellular level.
How to Do It
Application of antioxidants to corals has been trialed by Yudowski et al. (2018), who applied catechin, a plant-derived secondary metabolite and antioxidant, to heat-stressed Porites astreoides fragments and to the cnidarian model system Aiptasia pallida. They observed reduced ROS levels in host cells, which mitigated loss of algal symbionts from host tissues and prevented declines in their photosynthetic capacity. ROS-scavenging nanoparticles (RNP°) have also been developed for application in tumor suppression and anti-inflammation in model vertebrate organisms such as zebra fish (Vong et al., 2015, 2016) and more recently applied to coral larvae to mitigate thermally induced oxidative stress (Motone et al., 2018). RNP° are of a size that prevents interference with normal cellular redox reactions, such as the electron transport chain within cells, while still allowing for scavenging of ROS and mitigation of oxidative stress (Vong et al., 2015). When RNP° was added to thermally stressed Acropora tenuis larvae (without algal symbionts), ROS-induced oxidative stress proteins were absent compared to controls without the RNP° addition. Theoretically, other antioxidants can be trialed, and would need extensive testing on positive and negative effects at the cellular and whole-colony level. The committee could not find any published studies on the efficacy of iron addition at times of high-temperature stress to mitigate the effects of coral bleaching.
Current Feasibility
Addition of antioxidants to coral to mitigate ROS production under environmental stress is currently in the very early stages of testing feasibility, using cellular assays and testing on model cnidarian (including coral) species. The understanding of the effectiveness of this approach, although promising in early studies, is also rudimentary. It is therefore not currently feasible to implement these approaches without an extensive research and development stage that documents the benefits and any negative outcomes at the cellular, whole animal, and ecosystem levels. Subsequent investigations would also need to look at how the approach could be implemented at large scales with questions around effective delivery of the ROS-scavenging antioxidants as well as other chemical additions.
Potential Scale
Currently the scale of application of antioxidants has only been tested at the colony level, and the potential for application to reef-scale processes is unknown. The efficacy of the approaches at reef scale would be highly dependent on finding an appropriate and efficient delivery method to benefit coral during times of stress and increased internal ROS production. At temporal scales, provided the approach is shown to be effective, addition of the antioxidants and other chemicals would be required during early and peak periods of environmental stress, and potentially even following the stress events to help coral recovery.
Risks
The risks are currently unknown and would need to be assessed on each identified individual antioxidant or other chemical. While many of the antioxidants are naturally produced compounds, application of high concentrations may have detrimental impacts on organismal function. Hence, for reef-scale applications, baseline ecotoxicology assessments and safe dosing guidelines (LD50s) may need to be developed for each identified compound. A study that looked at the effect of iron addition to coral showed that the growth of the algal symbiont increased, with the effect of reducing coral calcification, likely due to reduced transfer of carbon to the coral host (Ferrier-Pagès et al., 2001). Stimulation of plank-tonic biomass at a large scale has been proposed as a way to draw down and sequester carbon dioxide (“iron fertilization”), and the potential risks that have been identified for this approach could apply to application in coral reef environments. The risks are that phytoplankton communities may quickly dominate and can reduce oxygen levels and other essential
nutrients, which would negatively affect other marine organisms and also lead to harmful algal blooms (NRC, 2015).
Limitations
Variations in responses by various coral species to the range of potential antioxidants limits knowledge on the effectiveness of a given application. The addition of catechin to control colonies that were not subjected to temperature stress during the experiments of Yudowski et al. (2018) resulted in loss of the symbionts. This indicates a potential for the antioxidant to damage normally functioning photosynthetic pathways due to the potential importance of ROS at certain levels for signaling and cellular processes. The response to catechin was also different between the coral P. astreoides and the sea anemone Aiptasia pallida. The other currently documented approach of using RNP° has been designed to avoid interference with normal cellular redox reactions, though limited understanding and the early stage of development, limits its current application for mitigation of coral stress in the field. Therefore, while addition of antioxidants may seem a promising and a future feasible option, much more work is required to understand the cellular processes mitigating ROS production and related cellular dysfunctions, the responses over many other taxa, and the ability to apply specifically to coral at scales that are effective.
Infrastructure
The infrastructure associated with antioxidants to mitigate cellular stress processes is focused on the experimental research and development phases. This includes laboratory and aquarium-related facilities where the efficacy of the approach can be tested. Testing is needed at the individual coral colony scale as well as for methods for upscaling the benefit of antioxidants or other chemicals to whole reefs or corals held within husbandry facilities, for which infrastructure requirements are currently unknown.
NUTRITIONAL SUPPLEMENTATION
What It Is
Nutritional supplementation of corals with carbon and other essential nutritional elements during periodic episodes of major stress events (e.g., bleaching that impacts their metabolic competence) represents an additional novel intervention strategy. Improved nutritional status of corals can provide increased resilience when faced with environmental stress
and condition corals in the longer term to disease and other abiotic and biotic challenges. Nutritional supplementation may compensate the coral host for compromised function of the algal symbiont cells during times of environmental stress. Within aquarium systems, supplementation of corals’ energy requirements through heterotrophic feeding is already an established and essential requirement to maintain health.
Benefit and Goals
Interventions that deliver greater nutritional supplementation to coral may improve their resilience during times of environmental stress by compensating for the lost energy resulting from algal symbiont dysfunction during bleaching events. The observed visual response of coral to stress is often a result of metabolic dysfunction at the cellular level, which is directly derived from disruption of the supplied essential nutritional elements (carbon, nitrogen, sulfur, phosphorus, and trace nutrients). Healthy zooxanthellae-containing corals acquire carbon both heterotrophically and photoautotrophically. Up to 60% of the corals’ requirements can be derived via predation on zooplankton, pico- and nanoplankton, dissolved organic matter, and suspended particulate matter while up to 90% can be obtained via photosynthetically fixed carbon translocated from zooxanthellae (Falkowski et al., 1984, 1993; Grottoli et al., 2006; Muscatine et al., 1981; Palardy et al., 2008). The underlying mechanisms of carbon capture within the coral holobiont are still not well understood, however, and likely differ greatly between different coral species with varying morphologies (Ferrier-Pagès et al., 2011). Recent studies have demonstrated that 70%-100% of daily carbon requirements are met through heterotrophic feeding and that corals can up-regulate feeding when photosynthesis is lower (Ferrier-Pagès et al., 2011; Grottoli et al., 2006; Houlbrèque and Ferrier-Pagès, 2009; Tremblay et al., 2015). This is especially important during periods of temperature stress when algal symbionts are lost from the coral tissues and corals increase heterotrophic feeding to compensate (Palardy et al., 2008; Tremblay et al., 2016). The endolithic communities of corals have also been reported to be an important route of carbon into the coral holobiont, with blooms of endolithic algae during a coral bleaching event partially supplementing lost carbon (Fine and Loya, 2002).
How to Do It
Nutritional sources include a range of zooplankton, picoplankton, and nanoplankton. The coral aquarium trade, research facilities, and
hobby aquarists routinely supplement the coral diet with a range of commercial feeds that include phytoplankton, rotifers, krill, and even pieces of shrimp, squid, or clams. However, there is currently no dedicated or robust assessment of an optimized coral diet supplementing nutrition to build coral resilience.
Current Feasibility
Nutritional supplementation of corals is mature for corals that are maintained in small-scale hobbyist tanks and larger-scale display and research aquarium facilities. Facilities that are focused on growing coral for outplanting onto reefs and that can provision the corals with the best nutritional competency will have important beneficial effects for survivorship and building resilience in coral populations. Optimized diets may also include probiotic microbial candidates (probiotic cocktails) or enhanced antioxidants that improve the fitness traits of coral. For in situ reef-wide applications, the approach is currently not feasible; work is required to develop improved coral-specific diets that can be delivered most efficiently for a wide number of coral taxa that can be differently constrained by polyp size and requirements of heterotrophic and autotrophic energy acquisition.
Potential Scale
The current scale of nutritional supplementation of corals is only in enclosed conditions at the hobbyist scale and small to large coral aquaculture/propagation facility levels. Operations at reef scale have not been undertaken to date and the potential risks and benefits not quantified. Wide-scale delivery could be achieved by drawing on expertise and approaches from the agricultural sector (i.e., fertilizer application can be considered nutritional supplementation of crops). Currently the approaches that would deliver an appropriate heterotrophic diet to coral in situ have not been developed. On a temporal scale, nutritional supplements would be applied during early and peak periods of environmental stress, and potentially even following the stress events to help coral recovery.
Risks
Ecosystem-level impacts include the effect of the addition of excess labile carbon, nitrogen, and phosphate into the reef environment on nutrient dynamics. Growth of species that more easily assimilate bioavailable
carbon may be stimulated (e.g., macroalgae), allowing them to outcompete the corals that are already compromised. The additional carbon may also drive reef microbial processes that shift the flow of carbon through the food webs.
Within the coral itself, both nutritional extremes and change in the ratios of nutrients can disrupt the symbiosis between corals and their algal partners, making them more susceptible to bleaching (Wooldridge, 2009a, 2009b). Recent studies have linked declines in coral health to specific nutrient sources and ratios (D’Angelo and Wiedenmann, 2014; Wiedenmann et al., 2013) and hence if the nutritional balance of supplied food sources is poorly matched to coral physiological responses, the addition of these nutrient sources may lower coral resilience to environmental perturbations. Negative impacts are largely attributed to increased N:P ratios. While particulate food and moderate levels of ammonium and phosphate tend to benefit coral health and thermal tolerance (Béraud et al., 2013; Ezzat et al., 2016; Shantz and Burkepile, 2014), high nitrate negatively impacts coral health and reduces thermal tolerance unless accompanied by a higher level of phosphorus (D’Angelo and Wiedenmann, 2014; Rosset et al., 2017; Shantz and Burkepile, 2014; Wiedenmann et al., 2013). Consideration of the internal nutrient budget of corals and its influence on coral physiology is essential in prospective work that promotes nutritional supplementation of corals.
Limitations
Effectiveness is limited by poor understanding of coral diets and nutritional requirements and limited knowledge of species-specific responses to different nutritional supplements. There is a requirement for extensive development of technology and infrastructure to deliver heterotrophic nutrients to corals in situ. Nutrient encapsulation and formulations are well established in other industries (e.g., animal food industries) and can potentially be adapted to a novel challenge in the marine environment. Nevertheless, the suitability of the nutritional supplementation requires extensive evaluation with well-designed laboratory and aquarium-based experiments.
Infrastructure
A research and development phase would be needed to optimize coral diets. Subsequent costs and infrastructure would be moderate due to existing technologies in other sectors that could easily be adapted. Therefore, research targeted at developing improved coral diets is required and
can draw on experience in other industries, including human and veterinary health and agricultural systems for improving the efficacy of supplementation. This can include developing diets with broad benefits to coral health, not only through nutritional supply but also through improved delivery of microbial symbionts (i.e., probiotics cocktails containing both algal and prokaryote communities) and other beneficial augmentation (e.g., antioxidants). The infrastructure needed to deliver heterotrophic coral diets at the scale of reefs at this stage is unknown without extensive feasibility testing and development. At the aquarium scale, these systems and approaches are already implemented.
This page intentionally left blank.