5
Responding to the Need for Spatial Thinkers
5.1 INTRODUCTION
On March 15, 2003, the Economist published an article with the provocative title of “The Revenge of Geography.” The thesis is that “… it was naive to imagine that the global reach of the internet would make geography irrelevant. Wireline and wireless technologies have bound the virtual and physical worlds closer than ever” (Economist, 2003, p. 19). The virtual world overlays and intersects with the real world in which we live. For example, Internet protocol (IP) addresses have a physical expression in the geographic location of the computer and its user. The geographic location of mobile devices can be determined through GPS. The committee would argue that the global reach of the Internet will make the understanding of space even more important. Access to and intelligent use of the virtual world will place a premium on spatial thinking in everyday life, in the workplace, and in science. To what extent is the American K–12 educational system responding to such needs by producing students who are at least familiar with, if not expert at, spatial thinking?
This chapter reviews the extent to which the educational system is meeting societal needs for spatial thinkers. Sections 5.2 and 5.3 show how the increasing importance of the information technology sector is generating demands for skilled workers with spatial skills and for citizens who can use spatial tools and technologies in their everyday lives. Sections 5.4 and 5.5 look at educational responses, first in terms of international comparisons of spatial skills of students and second in terms of the role of spatial thinking in standards-based education. Given the arguments in the four chapters in Part 1 of this report, in Section 5.6 the committee develops a position statement advocating the need to teach spatial thinking to every K–12 student.
5.2 THE INCREASING NEED FOR SPATIAL THINKING SKILLS
At the simplest level, the root metaphors for describing access to the virtual world of the Internet are spatial: portals, desktops, connectivity, traffic, speed, addresses, geocodes and geotags, cyberspace, navigation, and search. As the Economist argues, “Finding information relevant to a particular place, or the location associated with a specific piece of information, is not always easy.
This has caused a surge of innovation, as new technologies have developed to link places on the internet with places in the real world—stitching together the supposedly separate virtual and physical worlds” (Economist, 2003, p. 19). Geolocation systems match Internet addresses and physical locations. Thus, we are moving into a world of personal computing functionality (such as geo-enabled Personal Digital Assistants [PDAs] in car navigation systems [see Box 6.2] and cell phones with GPS functions), which provide us with and enable us to use spatial information. For example, Location Based Services (LBS), which link mobile devices (cell phones, PDAs, etc.) with GPS technology, allow for the tracking of people in geographical space and for the provision of real-time information to people as they move in space (Did you know that store X, only 50 yards away, has a sale on item Y?). That information would come from access to your recent purchasing patterns, your current location, and the proximity to a store offering similar goods. As Dobson and Fisher (2003, p. 52) pointed out, “LBS offers major benefits on the one hand and horrendous risks on the other.” In either case, the spatial thinking skills of the user are an essential prerequisite.
At a more fundamental level, there is an intersection between information technology (IT) and the idea of space. The intersection takes a variety of forms. It is estimated, for example, that 80 percent of all data can be given spatial coordinates and therefore spatialized. It is similarly estimated that 80 percent of all text documents contain geographical references (M. F. Goodchild, personal communication, 2003). Fabrikant and Buttenfield (2001) show that it is possible to spatialize various forms of knowledge and that the spatialization of mapping domains has become a topic of great interest in information science. The management of increasingly massive databases depends on data mining, a technique that draws extensively on spatial concepts, and visualization, an approach that is equally spatial in its foundations. The classic example of this convergence of spatialization and visualization is the analytic technique of multidimensional scaling (MDS), wherein measures of similarity are defined between objects in a data set (e.g., automobiles, books in a library, characters in a play). MDS attempts to create a space of a prescribed number of dimensions, and to find locations for objects in that space, such that the distances between pairs of locations in the space are as closely correlated as possible with the measures of similarity. The output of the program can be displayed graphically.
This convergence between concepts of space and IT means that, increasingly, problems will be posed in and solved within spatial frameworks (Goodchild, 2001). In turn, people will be presented with opportunities to and faced with the need to think spatially in everyday life (using MapQuest to generate driving directions), in the workplace (using a display system to route traffic or control aircraft), and in science (using the visual output of a data mining program).
5.3 PROVIDING SKILLED SPATIAL THINKERS
A strong component of the drive for K–12 educational reform since the 1989 Charlottesville Education Summit has been a concern about the capacity of the American workforce to compete successfully in a context defined by intense international competition in global markets and rapid technological change in the nature of the work process. These concerns are part of the rationale for two of the National Education Goals: Goal 3 (“all students will leave grades 4, 8, and 12 having demonstrated competency over challenging subject matter … and every school in America will ensure that all students learn to use their minds well, so that they may be prepared for responsible citizenship, further learning, and productive employment in our Nations’ modern economy”) and Goal 6 (“every adult American will be literate and will possess the knowledge and skills necessary to compete in a global economy and exercise the rights and responsibilities of citizenship”).
The thrust of the Secretary of Labor’s Commission on Achieving Necessary Skills (SCANS) report (U.S. Department of Labor, 1991, p. 2) was that “for most of this century, as this nation took its goods and know-how to the world, America did not have to worry about competition from
abroad. At home, the technology of mass production emphasized discipline to the assembly line. Today, the demands on business and workers are different. Firms must meet world class standards and so must workers.” The report emphasized the need to reform American education in order to foster “five competencies which, in conjunction with a three-part foundation of skills and personal qualities, lie at the heart of job performance today. These eight areas represent essential preparation for all students, both those going directly to work and those planning further education. All eight must be an integral part of every young person’s school life.” The five competencies included the productive use of resources, interpersonal skills, information, systems, and technology. The skills comprise basic skills (reading, writing, arithmetic and mathematics, speaking, and listening), thinking skills, and the diligent application of personal qualities. Taken together, these eight competencies and skills comprise the needed “workplace know-how.”
American employers are increasingly concerned about the knowledge and skills of high school graduates. They perceive a mismatch between their needs for a skilled, knowledgeable, and flexible workforce and the products of the K–12 education system. On-the-job training is expensive and disruptive to employers and workers alike. From the perspective of potential workers, the nature of work is changing. Not only are workers facing greater expectations in terms of knowledge and skills, but they are expected to participate in what is essentially a lifelong learning process. Knowledge and skills must be maintained, upgraded, and replaced. Thus, on average, American workers can expect to change employment seven times during a career. With each change come new demands—and opportunities—for work-related knowledge and skills. With each change comes the possibility of obsolescence.
At the heart of the necessary workplace skills are those of critical thinking. Halpern (1999, p. 74) identifies these as comprising (1) verbal reasoning, (2) argument analysis, (3) thinking as hypothesis testing, (4) using likelihood and uncertainty, and (5) decision making and problem solving. The SCANS (U.S. Department of Labor, 1991, p. 3) report argued that “employers and employees share the belief that all workplaces must ‘work smarter’” and, in the title of his report to the Russell Sage Foundation, Hunt (1995) posed the question: Will We Be Smart Enough? His subtitle, A Cognitive Analysis of the Coming Workforce, captures his approach to the question. Hunt linked cognitive theory, psychometric approaches, and demographic projections to analyze workforce needs over the next decades. His focus on cognitive skills (such as problem solving and flexibility) reflects the emergence of the concept of knowledge workers in the information economy. Halpern (1998) defines knowledge workers as people who can carry out multistep operations, manipulate abstract and complex symbols and ideas, efficiently acquire new information, and remain flexible enough to recognize the need for continuing change and for new paradigms for lifelong learning. Knowledge workers are central to the rapidly emerging IT sector of the U.S. economy. By some estimates, 60 percent of the American workforce can currently be classified as knowledge workers. Even if that is an overestimate, IT will increasingly dominate workforce needs over the next decades.
One segment of the IT market, the geospatial information and technology sector, is projected to have annual revenues of $30 billion dollars by 2005 (Gaudet and Annulis, under review), with a job growth rate in related professions of 10–20 percent between 2000 and 2010 (Gaudet et al., 2003). As Gaudet and Annulis (under review) noted, “there is a serious shortfall of professionals and trained specialists who can utilize geospatial technologies in their jobs. The growth of this market demands support of the education, training, and development of geospatial professionals and specialists.” Funded by NASA, the National Workforce Development Education and Training Initiative has developed a Geospatial Technology Competency Model (http://geowdc.com/assessment/) (see Table 5.1). It is built on an industry definition: Geospatial technology is an information technology field of practice that acquires, manages, interprets, integrates, displays, analyzes, or otherwise uses data focusing on the geographic, temporal, and spatial context. In effect, the
TABLE 5.1 The Geospatial Technology Competency Model
Technical Competencies |
Business Competencies |
Ability to assess relationships among geospatial technologies Cartography Computer programming skills Environmental applications GIS theory and applications Geology applications Geospatial data processing tools Photogrammetry Remote-sensing theory and applications Spatial information processing |
Ability to see the “big picture” Business understanding Buy-in, advocacy Change management Cost-benefit analysis, Return on Investment Ethics modeling Industry understanding Legal understanding Organization understanding Performance analysis and evaluation |
Technical writing Technological literacy Topology |
Visioning |
Analytical Competencies |
Interpersonal Competencies |
Creative thinking Knowledge management Model building skills Problem-solving skills Research skills Systems thinking |
Coaching Communication Conflict management Feedback skills Group process understanding Leadership skills Questioning Relationship building skills Self-knowledge, Self-management |
NOTE: Core competencies are shown in bold. SOURCE: http://geowdc.com/assessment/. |
Geospatial Technology Competency Model establishes a link between competencies—the knowledge, skills, and abilities that an individual needs to do a job—and roles, which are groupings of work-related competencies. Many of the technical and analytical competencies listed in the model are directly related to the process of spatial thinking.
In short, therefore, workforce demands are changing; those demands can be met only if the K–12 education system produces graduates with the requisite skills and knowledge, with a commitment to lifelong learning, and with flexibility to adapt to change. Central to changing workforce needs are knowledge workers for the rapidly growing IT sector. Central to the IT sector and many other sectors is spatial thinking. To what extent does the K–12 educational system generate graduates with these spatial thinking skills? One answer can be found in the most recent international comparative survey of mathematics and science performance.
5.4 THE 1999 TRENDS IN INTERNATIONAL MATHEMATICS AND SCIENCE STUDY (TIMSS)
The Trends in International Mathematics and Science Study (formerly known as the Third International Mathematics and Science Study) is the international parallel to the National Assessment of Educational Progress. Sponsored by the U.S. Department of Education through the Na-
tional Center for Education Statistics (NCES), TIMSS compares the performance of American students (at the end of the eighth grade) with those of other countries in five major content areas of mathematics and six content areas in science. TIMSS has been offered in 1995 and 1999 (the 2003 results are not yet available). Therefore, TIMSS provides the most reliable and comprehensive assessment of trends in student achievement from an international perspective.
The TIMMS 1999 results do not speak directly about spatial thinking as such. However, there are content areas in which performance would seem to be directly related to skill in spatial thinking. In the case of mathematics, performance in geometry is defined as understanding points, lines, planes, angles, visualization, triangles, polygons, circles, transformations, symmetry, congruence, similarity, and constructions (Mullis et al., 2000, p. 93). Although the average U.S. scale score for geometry was 473 and not statistically significantly different from the international average of 487 (range 575–335), U.S. students did rank 27 out of 38 countries. Thus, “the United States performed significantly above the international average in fractions and number sense; data representation, analysis, and probability; and algebra. In contrast, however, it performed similarly to the international average in measurement and geometry (a shift in ranking from 16th in data representation, analysis, and probability to 27th in geometry)” (Mullis et al., 2000, p. 94).
U.S. students showed a small but not statistically significant increase in achievement between 1995 and 1999. As the NCES (2000b, p. 108) report notes: “Changes in average achievement at a national level are not easy to bring about and inevitably take place over several years. Amending official curricula, producing relevant supporting resources, and changing teacher practice all take time, even under the most favorable conditions.”
In the case of science, two content areas—Earth science (includes Earth features, Earth processes, and Earth in the universe) and physics (includes physical properties and transformations, energy and physical processes, and forces and motion)—appear to have strong connections to spatial thinking. With the notable exception of physics, the average achievement of U.S. students was significantly higher than the international average. The ranking of U.S. students ranged from 13th out of 38 in the case of scientific inquiry and the nature of science to 20th for physics. U.S. students ranked 16th out of 38 in achievement in Earth science. For the four areas for which trend data are available (Earth science, life science, physics, and chemistry), there was no significant difference between 1995 and 1999.
Given the importance of science and mathematics achievement and the significant investment in those areas of education, the TIMSS results are neither impressive nor satisfactory. While it is true that changing average levels of achievement is difficult, current educational policy, as exemplified by the No Child Left Behind Act of 2001 (Public Law 107-110), is committed to fostering academic achievement in core subjects (defined as English, reading or language arts, mathematics, science, foreign languages, civics and government, economics, arts, history, and geography). For at least three of the TIMSS content areas (geometry, physics, and Earth science), achievement is related to competence in spatial thinking, and to the extent that spatial thinking is taught with adequate supports, the committee believes that achievement in mathematics and science understanding will improve. However, that improvement will come about only if the educational system focuses its attention on spatial thinking. To what extent is that happening?
5.5 SPATIAL THINKING IN THE NATIONAL EDUCATION STANDARDS
5.5.1 The Role of Spatial Thinking in the Mathematics and Science Standards
In the current educational environment one important place to look for attention to spatial thinking is in the educational standards for various disciplines. These discipline-based standards, developed in the middle to late 1990s, provide statements of what K–12 students should know,
understand, and be able to do; they are intended to serve as a basis for the development of curricula, assessment procedures, teacher-training programs, and supplementary instructional material.
The committee focused on two sets of standards:
-
Principles and Standards for School Mathematics, prepared by the National Council of Teachers of Mathematics in 2000. This is an update of the first-ever set of standards, those published for mathematics in 1989.
-
National Science Education Standards, prepared by the National Research Council in 1996.
Several sets of standards were examined, including those for geography (Geography Education Standards Project, 1994), but the mathematics and science standards offer a direct connection to spatial thinking and reasoning and they are fundamental to the process of education and to the idea of a technologically skilled workforce. (Spatial thinking is integral to geography as a discipline and, thus, figures prominently throughout the national geography standards. The first 3 of the 18 standards and all five of the sets of geographic skills are a primer in thinking spatially about geographic data; see Box 5.1).
As is the case for most education standards, these two sets of standards are organized in terms of intellectual themes with progressively more challenging standards of performance established for different grade levels along each theme. For example, the science standards are built around eight intellectual categories: unifying concepts and processes in science, science as inquiry, physical science, life science, Earth and space science, science and technology, science in personal and social perspectives, and the history and nature of science. For each category there is a content standard and “as a result of activities provided for all students in those grade levels, the content of the standard is to be understood or certain abilities are to be developed” (NRC, 1996, p. 6). In the case of the first category, there is no distinction by grade level; for the other seven categories, understanding is organized into three grade clusters: K–4, 5–8, and 9–12. The eight standards are to be used as a whole in order to achieve scientific literacy.
There are two questions about the relationship between spatial thinking and the sets of content standards: (1) Are the basic tenets of spatial thinking an explicit part of the expectations established by various standards? (2) Are spatial thinking concepts implicitly contained within the standards? To answer these questions, the committee considers the two sets of standards in sequence, beginning with mathematics because it has been in place since 1989 in its original form.
5.5.2 Spatial Thinking and the Principles and Standards for School Mathematics
The mathematics standards are built around five content standards—number and operations, algebra, geometry, measurement, and data analysis and probability—and five process standards—problem solving, reasoning and proof, communication, connections, and representation (NCTM, 2000). For each standard, there are two to four statements of what students should be able to do as a consequence of instruction, with specific expectations keyed to grade levels K–2, 3–5, 6–8, and 9–12. These expectations are summarized in Table 5.2.
In Table 5.2, only the geometry standard uses the language of spatial thinking (two and three dimensions, shapes, relationships, locations, spatial reasoning, etc.). However, in reading the detailed discussions, grade by grade, for each of the ten mathematics standards, it is clear that spatial thinking pervades and permeates the detailed articulation of what is expected of students. There are explicit references to spatial concepts in the geometry, measurement, data analysis and probability, and representation standards. Although the term spatial thinking is not used explicitly in the text of Table 5.2, the underlying concepts are implicit throughout. Thus, an understanding of spatial relations is presumed but never discussed explicitly. For many of the statements in Table 5.2,
BOX 5.1 As the opening definition of geography suggests, the discipline and spatial thinking are inextricably interwoven: “Geography is the science of space and place on Earth’s surface” (Geography Education Standards Project, 1994, p. 1). The definition is extended to argue that “geography means a sensitivity to location, to scale, to movement, to patterns, to resources and conflicts, to maps and geographics” (Geography Education Standards Project, 1994, p. 18). The standards are built around three components: subject matter (in the form of 18 content standards), skills (in the form of five sets of skills), and perspectives (in the form of two major perspectives [the spatial and the ecological] and two supporting perspectives [the historical and the economic]). The first three content standards are grouped under the idea of “the world in spatial terms”: Standard 1: How to use maps and other geographic representations, tools, and technologies to acquire, process, and report information from a spatial perspective Standard 2: How to use mental maps to organize information about people, places, and environments in a spatial context Standard 3: How to analyze the spatial organization of people, places, and environments on Earth’s surface Geographic skills provide the necessary tools and techniques for students to think geographically (or, as is clear, think spatially). This set of five skills makes an explicit link among three elements: the process of doing science, the process of problem solving, and the process of thinking spatially. The five skill sets are (1) asking geographic questions, (2) acquiring geographic information, (3) organizing geographic information, (4) analyzing geographic information, and (5) answering geographic questions. Thus, at the end of the fourth grade, students would be expected to prepare maps, graphs, tables, and diagrams to display geographic information (skill set 3). They would be able to analyze Earth’s surface in terms of its spatial elements of point, line, area, and volume (Standard 3). They would be able to locate Earth’s continents in relation to each other and to principal parallels and meridians (Standard 2). They would be able to use a map grid (e.g., latitude and longitude or alphanumeric system) to answer the question—What is this location? (Standard 1). The cumulative structure of increasingly demanding standards, skills, and perspectives is aimed at a high school graduate who is geographically informed, seeing meaning in the arrangement of things in space; seeing relations between people, places, and environments; using geographic skills; and applying spatial and ecological perspectives to life situations. Spatial thinking underpins the intellectual structure of the geography standards. Interestingly, however, these standards are also stand-alone. Although there is a direct connection with the discipline of history through one content standard, there is no explicit connection with either the mathematics or the science standards. Given the way geography is taught in elementary school—as part of social studies—there is a very low probability that students would enjoy the benefits of coordination between, say, mathematics and geography teaching and learning. The geography standards demonstrate the possibility and power of infusing spatial thinking into a discipline. They also show the need for a coordinated approach to spatial thinking standards across the curriculum. Ironically, they illustrate the rapid change in technology, as well: GIS as a support system for geographical thinking was relegated to an appendix rather than being integrated into the structure of the geography standards. The geography standards, therefore, exemplify the possibility of developing standards for spatial thinking across the curriculum. |
spatial concepts could be applicable. In numbers and operations, ways of representing numbers could include a number line, which is a spatialization. In communication, spatializations in graphs could support the process. In data analysis, graphs could be used. However, in this most fundamental statement of what students should know and be able to do in mathematics, space and spatial thinking do not play a prominent role.
The geometry standard provides explicit connections to the concepts of spatial thinking. In fact, the opening paragraph of the broad discussion of geometry states:
TABLE 5.2 Table of Standards and Expectations for School Mathematics
Number and operations |
|
Algebra |
|
Geometry |
|
Measurement |
|
Data analysis and probability |
|
Problem solving |
|
Reasoning and proof |
|
Communication |
|
Connections |
|
Representation |
|
Spatial visualization—building and manipulating mental representations of two-and three-dimensional objects and perceiving an object from different perspectives—is an important aspect of geometric thinking. (NCTM, 2000, p. 41)
In many ways, this statement parallels part of the committee’s definition of spatial thinking. The discussion of the standard goes on to make the point that “geometric modeling and spatial reasoning offer ways to interpret and describe physical environments and can be important tools in problem solving” (NCTM, 2000, p. 41).
The second and third parts of the geometry standard, which refer to the specification of location and the use of transformations, are strongly connected to the spatial concepts discussed in Chapter 2. The importance of spatial concepts is clear from the detailed discussion of the standard at each grade level. For example, a succession of activities that require spatial thinking can be found in progressing through the first two grade groups.
-
Grades K–2
-
Describe, name, and interpret relative positions in space and apply ideas about relative position.
-
Describe, name, and interpret direction and distance in navigating space and apply ideas about directions and distance.
-
Find and name locations with simple relationships such as “near to” and in coordinate systems such as maps.
-
Grades 3–5
-
Describe location and movement using common language and geometric vocabulary.
-
Make and use coordinate systems to specify locations and to describe paths.
-
Find the distance between points along horizontal and vertical lines of a coordinate system.
In light of one of the charges to the committee—to address the utility of GIS in supporting education—there are two interesting aspects to the mathematics standards.
The first is contained in the representation standard, which calls for the ability to “use representations to model and interpret physical, social, and mathematical phenomena” (NCTM, 2000, p. 67). The detailed explication of this standard asks what a representation should look like and what
the role of the teacher should be in developing representations. Thus, the introductory explanation of the representation standard for grades 9–12 begins:
If mathematics is the “science of patterns” (Steen, 1988), representations are the means by which those patterns are recorded and analyzed. As students become mathematically sophisticated, they develop an increasingly large repertoire of mathematical representations and the knowledge of how to use them productively. This knowledge includes choosing specific representations in order to gain particular insights or achieve particular ends. (NCTM, 2000, p. 360)
In one respect, the mathematicians have an even broader concept of representation than is embodied in the committee’s approach to spatial thinking. For example, their view includes the discussion of numbers as representations. GIS is a system for generating multiple representations of spatial information, especially numerical information.
Second, the mathematics standards address the role of technology in the principles that serve as a preface to the standards and cover such matters as equity, assessment, and teaching. The technology principle states: “Technology is essential in teaching and learning mathematics; it influences the mathematics that is taught and enhances students’ learning” (NCTM, 2000, p. 11). In the detailed discussion of this principle, several points have bearing on the committee’s consideration of GIS as a support for spatial thinking: technology enhances mathematics learning, technology supports effective mathematics teaching, and technology influences what mathematics is taught.
Spatial thinking is, therefore, very much part of the mathematics standards. It is central to one content standard and incorporated in several others. The idea of GIS as a support system is implicit in one process standard, and the concept of a support system is endorsed in one of the principles—“describe particular features of high-quality mathematics education” (NCTM, 2000, p. 11). To what extent is this the case for the science standards?
5.5.3 Spatial Thinking and the National Science Education Standards
The relationship of the science standards to the basic concepts of spatial thinking is by no means as explicit as it is in the mathematics standards (NRC, 1996). A detailed reading of the science standards suggests that there is spatial thinking and spatial reasoning content, but neither phrase appears in the text, nor are the concepts addressed explicitly and systematically.
The science standards are similar in structure to those for mathematics. The one exception is that while the mathematics standards speak of “standards and principles,” the science standards broadly apply the concept of standards to every element of the educational system that touches the student-teacher relationship. So in the science standards there are standards for teaching, assessment, teacher preparation, and content, while the mathematics standards handle many of these topics in the principles.
The science content standards contain six basic themes, for which standards are elaborated in each of three grade intervals (K–4, 5–8, and 9–12). These themes are unifying concepts and processes; science as inquiry; physical science, life science, Earth and space science; science and technology; science in personal and social perspective; and history and nature of science.
The content standards for physical, life, Earth, and space sciences build on a theme associated with spatial thinking. This theme is one of an orderly progression from naming and locating objects, to placing objects in relationship to each other, naming those relationships, and then progressing to explanations of the spatial and functional structure of objects and their relationships. This parallels the discussion in Chapter 3 of the historical process that astronomy has taken from first locating and naming objects in the sky to inferring the structure and evolution of the universe. These steps are recapitulated in the Earth science standard, which begins with finding and describing Earth materials and culminates with the origin and evolution of the Earth system at level 9–12, including
explicitly in level 5–8 the spatial structure of the Earth system. In the life sciences standard a similar constructivist approach culminates at 9–12 with an understanding of matter, energy, and organization of living systems, progressing in grades 5–8 through the study of structure and function in living systems.
The science content standards necessarily reflect spatial thinking and reasoning in an implicit way because many of the objects of study for science exist in the real world. Thus, it is necessary to place things in that world, to speak to their relationships, and to make inferences about their structure and function. The question of how an explicit focus on spatial thinking might enhance that process of study remains unanswered in the science standards, with one possible exception in the program standards for the design and implementation of science programs at the school and district levels. Standard C of the program standards states that “the science program should be coordinated with the mathematics program to enhance student use and understanding of mathematics in the study of science and to improve student understanding of mathematics” (NRC, 1996, p. 214).
The discussion speaks to the value of mathematics, broadly referring to its use primarily in the display and analysis of data. While the science program standard implies a two-way street between mathematics and science, the narrative states:
Science requires the use of mathematics in the collection and treatment of data and in the reasoning used to develop concepts, laws, and theories. School science and mathematics programs should be coordinated so that students learn the necessary mathematical skills and concepts before and during their use in the science program. (NRC, 1996, p. 214)
This suggests that mathematical knowledge is a precondition for the development of scientific inquiry. Perhaps if spatial thinking is important to science education, there is a sense that students may have learned it as part of mathematics before they are expected to apply it in learning science.
Spatial thinking is, therefore, only an implicit but nevertheless essential part of the process of doing science, as articulated in the science standards. It is central to science, but it is not explicitly presented as part of the responsibility of science educators in fostering scientific literacy in K–12 students. To what extent are the two sets of standards coupled such that the prerequisite knowledge and skills are available to students?
5.5.4 The Role of Spatial Thinking in the Coupling Between the Mathematics and Science Standards
Several questions arise from this overview of the national standards for mathematics and science. Where exactly in the course of K–12 standards-based education are the fundamental concepts of space, representation, and spatial reasoning taught, exercised, and developed? Who—as in teachers of which school subject(s)—is responsible for ensuring that students develop increasing mastery of these ideas? Is this mastery available for transfer across the curriculum?
As noted above, the mathematics standards are constructivist, building to higher levels of abstraction as the student progresses through the grades to the level of understanding and competency expected of a graduating senior. Chapter 3 of the Principles and Standards for School Mathematics provides an integrated view of the progression of understanding and competency for all ten mathematics standards. Figures 5.1 and 5.2 are excerpts from two parts of the geometry standard: (1) specify locations and describe spatial relationships using coordinate geometry and other representational systems, and (2) use visualization, spatial reasoning, and geometric modeling to solve problems. These two parts are closely tied to concepts of space, representation, and spatial reasoning. Each of these parts has clearly stated outcomes with respect to mathematical understanding and contains clear examples of their application to real-world problems.
Chapters 4 through 7 of the mathematics standards describe how the goals are accomplished in
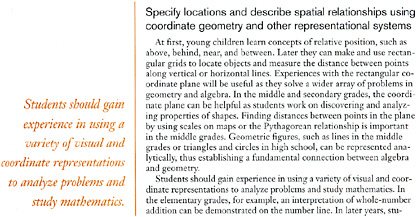
FIGURE 5.1 Specify locations and describe spatial relationships using coordinate geometry and other representational systems. SOURCE: NCTM, 2000. Reprinted with permission from Principles and Standards for School Mathematics, copyright 2000 by the National Council of Teachers of Mathematics. All rights reserved.
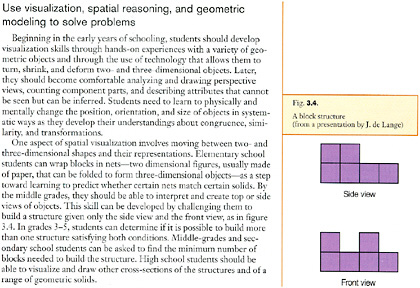
FIGURE 5.2 Use visualization, spatial reasoning, and geometric modeling to solve problems. SOURCE: NCTM, 2000. Reprinted with permission from Principles and Standards for School Mathematics, copyright 2000 by the National Council of Teachers of Mathematics. All rights reserved.
four developmental levels (pre-K–2, 3–5, 6–8, and 9–12). The progression for the geometry standard starts with the identification of points and coordinate systems, clearly oriented toward maps and other graphic representations, and the visualization of two- and three-dimensional objects. These basic concepts are developed in the corresponding sections of grade levels K–2 (Figure 5.3) and 3–5 (Figure 5.4) standards. In Figure 5.3 the idea of placing things in space begins a process of
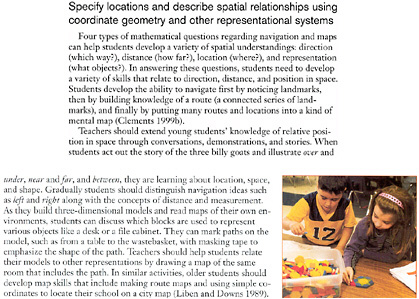
FIGURE 5.3 Specify locations and describe spatial relationships using coordinate geometry and other representational systems. SOURCE: NCTM, 2000. Reprinted with permission from Principles and Standards for School Mathematics, copyright 2000 by the National Council of Teachers of Mathematics. All rights reserved.
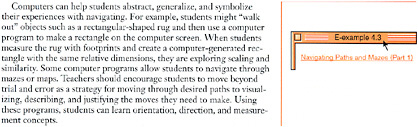
FIGURE 5.4 Specify locations and describe spatial relationships using coordinate geometry and other representational systems. SOURCE: NCTM, 2000.
constructing the ability to deal with more complicated spatial concepts that grows to ideas of distance and direction, part of a progression of increasingly complicated spatial concepts. In Figure 5.4 not only are these concepts developed further, enabling navigation for example, but also basic computer technology is used to support and enhance the learning process.
In Figure 5.5 the focus is on developing spatial thinking as a skill in young learners, explicitly in terms of navigation and visualization. By the time students are in grades 3–5, the geometry standard calls not only for the building of additional spatial thinking skills (Figure 5.6), but also for the teacher to explicitly tie those skills to other areas of mathematics and to other disciplines such as science.
How well is the mathematicians’ call for making ties to other disciplines reflected in and supported by the science standards? The simple concepts of position and shape (Figures 5.3 and 5.4) are nicely mirrored in the science standards. The science standards provide a guide to the specific science content at each grade level (K–4, 5–8, and 9–12) for each area of science covered (physical, life, Earth, and space). The content guide for the physical science standard for grades K–4 is shown in Figure 5.7. The sections on the physical properties of objects and materials and the position and motion of objects both use the spatial concepts being developed in the mathematics standard (Figure 5.3). Objects have position and shape in the science standard. In the geometry standard the concepts of position and shape are explicitly taught and reinforced. Similarly there are connections to other parts of the mathematics standards, and therefore the connection between the mathematics and science standards seems clear. At these early grades, the assumptions on the part of the writers of the science standards that mathematics will provide a prerequisite basis of knowledge that can be drawn on by science teachers and students seems appropriate.
Given this close connection between the science and mathematics standards based on similar words and concepts in the early grades, is this connection maintained in the higher grade levels?
Figures 5.8 and 5.9 contain the content guide to the physical science and the Earth and space science standards, respectively, for grade levels 9–12. The physical science standard focuses on atomic structure and the complex geometry of molecules, and the role of shape in chemical reactions (Figure 5.8). The Earth and space science standard talks about how the heating of Earth’s surface drives the ocean currents and winds (Figure 5.9). Achieving the understanding called for in each of these science content areas would be greatly enhanced by abilities in spatial representation, visualization, and spatial reasoning. Both sets of science concepts contain critical scientific content that requires the ability to visualize structures and to keep in mind complex physical relationships that vary over space and time.
For example, understanding how the energy of the Sun drives oceanic currents and atmospheric winds is complicated. In particular, the poleward transport of energy in both the atmosphere and the ocean is modified by the rotation of Earth. Understanding the space-time dynamics requires an ability to take concepts derived from a two-dimensional treatment of rotating frames of reference and adding the idea of the Coriolis effect to explain the more complex motion on the surface of a sphere that is required to explain phenomena such as the trade winds.
The geometry standard for middle school and high school requires students to “specify locations and describe spatial relationships using coordinate geometry and other representational systems” and to engage in “visualization, spatial reasoning, and geometric modeling” (Figures 5.10 and 5.11). The geometry standard does not appear to be developing the associated spatial thinking concepts in a fashion that is tightly coupled to the requirements implicit in the science standards for the parallel grades.
The expectation “specify locations” is moving into formal Euclidean geometry, a logical outcome for the geometry standard (Figure 5.12). There is some development of the concepts of coordinate systems on spheres and polar coordinates (Figure 5.13), which could be drawn upon by a science teacher in the Earth science activity relating to winds and ocean currents. The understanding of coordinate systems could be used to facilitate the projection of the three-dimensional sphere into two dimensions, but the science teacher would have to add the complicating factors of thinking about what is happening in a rotating coordinate system, something not addressed in the geometry standard.
Similarly, the “visualization” sections of the geometry standard for middle and high school students are not providing content that ties to the science standards. The standards for these grades emphasize networks (Figure 5.14) and the concept of perspective. In grade levels 6–8 there is additional work on two- and three-dimensional representations. All three concepts—networks, perspective, and representation—are important domains of knowledge for students to learn and
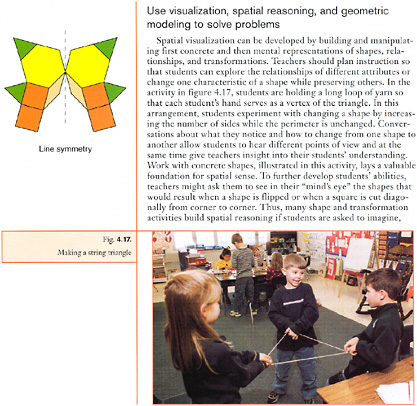
could be applied to science examples. However, in the specific case of winds and currents, only the content on representations appears relevant, and the development of an understanding of rotating frames of reference appears to be left to the science teacher.
As part of the grades 9–12 experience, the physical science standard involves developing an understanding of the geometry of molecules. An examination of the geometry standard (Figure 5.14) for the corresponding grade grouping does not yield any material that is closely connected to the kind of spatial thinking required for understanding the examples of molecules. However the geometry standards for pre-K–2 and grades 3–5 do contain material useful in developing spatial
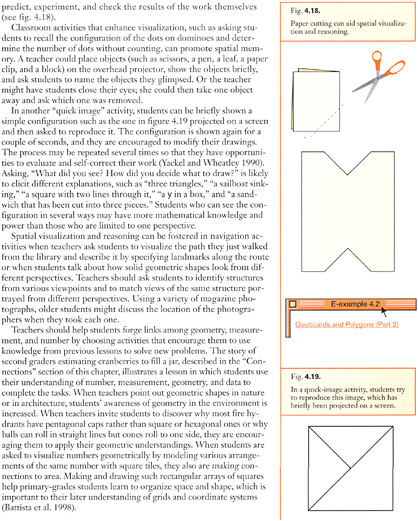
FIGURE 5.5 Use visualization, spatial reasoning, and geometric modeling to solve problems: developing spatial thinking. SOURCE: NCTM, 2000. Reprinted with permission from Principles and Standards for School Mathematics, copyright 2000 by the National Council of Teachers of Mathematics. All rights reserved.
thinking skills that could be used to understand three-dimensional molecular structures. Specifically, the visualization standard (Figure 5.6) for grades 3–5 has a focus on building up three-dimensional structures from blocks. In the pre-K–2 standards, students are asked to apply transformations and to use symmetry to analyze mathematical situations, skills that could again be useful in describing molecular structures. However, in sharp contrast to the strong links between K–4 mathematics and science, the necessary concepts in geometry are not introduced concurrently with science content that might use them. Therefore, the tight coordination of simple spatial concepts in the early years is not retained through high school.
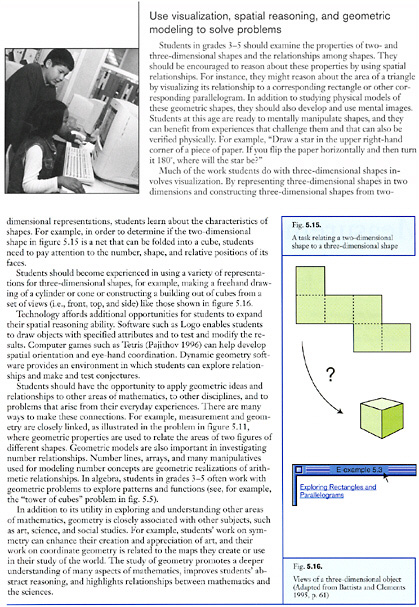
FIGURE 5.6 Use visualization, spatial reasoning, and geometric modeling to solve problems: building additional spatical thinking skills. SOURCE: NCTM, 2000. Reprinted with permission from Principles and Standards for School Mathematics, copyright 2000 by the National Council of Teachers of Mathematics. All rights reserved.
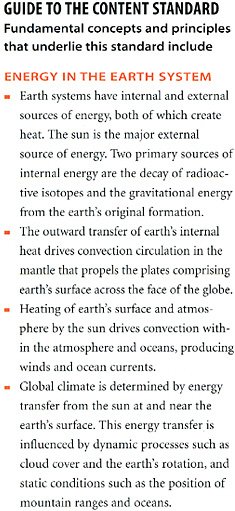
FIGURE 5.9 Guide to the content standard for Earth science in grades 9–12. SOURCE: NRC, 1996.
The lack of connection takes two forms. In the first case, that of grades 9–12 chemistry, the spatial thinking concepts that would allow the student to grasp the structure of atoms and molecules are introduced well ahead of need; therefore both students and teachers may not recall the relevant material from earlier grades. In the second case, that of the Earth and space science standard, other than the discussion of coordinate geometry, the committee could not find anything in the mathematics standards that supported the complex spatial thinking process associated with the description of the motion of fluids (wind and ocean currents) on a rotating sphere. It is interesting to note that the examples and explicit ties from the mathematics to the science standards that were so noticeable for early education (Figure 5.4) have largely disappeared.
There are several conclusions about the coupling of the use of spatial thinking and reasoning in the mathematics and science standards. First, there is a close connection between the mathematics (pre-K–2 and 3–5) and the science standards (K–4) in early education. Second, the development of
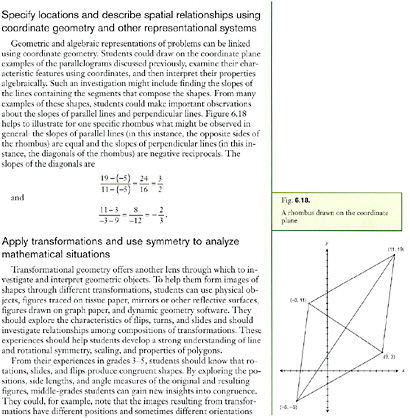
FIGURE 5.10 Specify locations and describe spatial relationships using coordinate geometry and other representational systems. SOURCE: NCTM, 2000. Reprinted with permission from Principles and Standards for School Mathematics, copyright 2000 by the National Council of Teachers of Mathematics. All rights reserved.
spatial thinking and reasoning in the early years is, in the mathematics standards, aided by computer-based support systems. Third, this close coupling is not present during the grades 9–12 experience. Specifically, the science standards continue to presume, but do not make explicit, the use of spatial thinking and reasoning. Further, the presumed spatial thinking skills are more sophisticated than those being emphasized in the mathematics standards. Fourth, higher-level ability for spatial thinking is central to many key science education outcomes such as the analysis of situations in rotating frames of reference. Finally, the science standards seem to presume a very sophisticated skill set in spatial thinking, reasoning, and representation, and it is unclear where in the education system that skill set has been developed. To the extent that spatial thinking skills are explicitly taught, the process occurs under the rubric of geometry, which is only one of ten standards that are to be met by mathematics teaching and learning. Therefore, there is currently no significant, systematic treatment of spatial thinking as part of standards-based instruction in the United States.
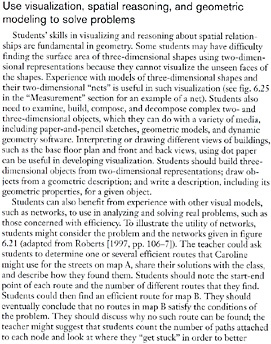
FIGURE 5.11 Use visualization, spatial reasoning, and geometric modeling to solve problems. SOURCE: NCTM, 2000. Reprinted with permission from Principles and Standards for School Mathematics, copyright 2000 by the National Council of Teachers of Mathematics. All rights reserved.
5.6 SPATIAL THINKING AND EDUCATION FOR THE NEXT GENERATION
Spatial thinking is a pervasive and powerful way of thinking that operates across the sciences, social sciences, and even the humanities. For example:
… chemical education demands so much from students. It requires them to understand abstract theories (sometimes two to explain one phenomenon!), to have mathematical skills, to have experimental skills, to be able to communicate orally and in writing, and to visualize in three dimensions given information in two dimensions. And that is just the start. (Molecular Visualization in Science Education Workshop Advisory Board, 2001, p. iv)
Spatial thinking underpins many of the standards documents, yet it is not explicitly mentioned in any of them. Spatial thinking is presumed in many if not all of the sets of national K–12 standards. Despite its apparent predominance in geometry, it is not limited to any one discipline (Box 5.1).
Spatial thinking is the start of successful thinking and problem solving. Skill in spatial thinking is presumed throughout the K–12 curriculum but is formally and systematically taught nowhere. This leads to an educational blind spot. Formal, systematic instruction in spatial ideas is not part of the mainstream educational program. Therefore, although many of the general concepts under the rubric of spatial thinking are not novel, the committee believes that spatial thinking is under appreciated and under instructed in a systematic and coordinated way.
The committee’s experience in reviewing the literature and examining the curriculum leads to a strong recommendation that we remedy the blind spot in the educational system by infusing and integrating diverse spatial thinking activities throughout the pre-college curriculum. Students could, for example, explore perspective in art; the perfect triangle in geometry; three-dimensional model-
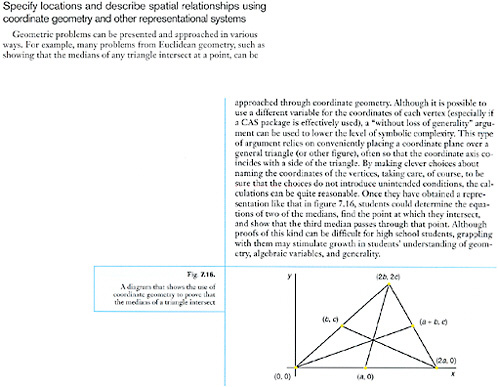
FIGURE 5.12 Specify locations and describe spatial relationships using coordinate geometry and other representational systems. SOURCE: NCTM, 2000. Reprinted with permission from Principles and Standards for School Mathematics, copyright 2000 by the National Council of Teachers of Mathematics. All rights reserved.
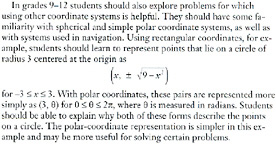
FIGURE 5.13 Geometry Standard: Specify locations and describe spatial relationships using coordinate geometry and other representational systems. SOURCE: NCTM, 2000. Reprinted with permission from Principles and Standards for School Mathematics, copyright 2000 by the National Council of Teachers of Mathematics. All rights reserved.
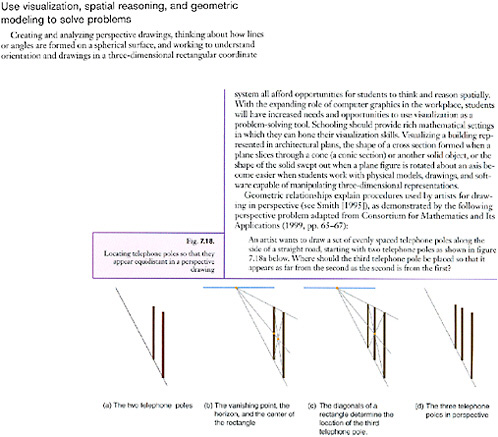
FIGURE 5.14 Use visualization, spatial reasoning, and geometric modeling to solve problems. SOURCE: NCTM, 2000. Reprinted with permission from Principles and Standards for School Mathematics, copyright 2000 by the National Council of Teachers of Mathematics. All rights reserved.
ing in biology, chemistry and physics; and multidimensional reasoning in design aesthetics, literature, and psychology. They should have an opportunity to see connections between the use of graphs in economics and phase diagrams in physics. They should be able to understand the connection between changes in point of view as a function of degree of rotation in the horizontal and vertical planes. They should be able to practice skills in paper-and-pencil mapping, physical model building, and computer representation.
Teaching about spatial thinking is of particular significance now because, on the one hand, the capacity to support spatial thinking is increasing through the prevalence, power, and opportunities available from computers and software and, on the other hand, there is an increasingly urgent need to make sense of the increasing volumes of spatialized or spatializable data that are readily available.
Spatial thinking is also significant now because of changes in the process of education and in the ways in which we characterize knowledge. Increasingly, teachers are adopting the inquiry
approach, which leads to fundamental changes in the relationship between teacher and student. The teacher becomes a facilitator or guide for the student’s own process of exploration, discovery, and understanding. The teacher relinquishes the role of expert authority and instead models and supports the learning process of students. Learning is no longer the accumulation of a specific body of knowledge or the memorization of correct answers—it is a process of exploration and discovery driven by curiosity (the students in Bruner’s study described in Chapter 1 [Bruner, 1959]). At the same time, there is a premium on connectivity and interdisciplinarity. Despite the power of the disciplinary approach to knowledge, real-world problems and the challenges of the workforce transcend traditional disciplinary boundaries. Multidisciplinary, collaborative approaches to problems are increasingly important. Again, spatial thinking is integral to these changes because it is a fundamental means of inquiry and because it connects across and adds coherence to the curriculum.
Therefore the committee believes that (1) we must recognize that spatial thinking is a fundamental and necessary mode of thought applicable across the life span in everyday life, in work situations, and in science; (2) we must foster a generation of students who are spatially literate; and (3) we must facilitate the transfer of generalizable spatial thinking skills across domains of knowledge in the K–12 curriculum, thus enhancing learning across the curriculum.
Spatial thinking can and should be taught in American schools. Students need formal training in specific spatial thinking skills (e.g., using point and cross-sectional data to create a three-dimensional model in anatomy or geology). There are many such spatial thinking skills, some of which may be specifically “tuned” to the needs of a particular disciplinary community.
We also need an educational process that leads to a fundamental understanding of spatial thinking in general, something that is more than a set of specific skills tailored to a particular discipline or school subject. Currently there are no standards for how we should think or learn spatially and no standards for how spatial thinking can be taught and assessed.
Recommendations for change in education encounter many problems, not the least of which is the understandable resistance of teachers who face increasing public demands for accountability on the one hand and demands for depth of coverage in specified knowledge domains on the other. Examples of such demands are those present in the No Child Left Behind Act of 2001 (Public Law 107-110), which mandates coverage of core areas of knowledge and regular assessment of student achievement in those areas. Adding another set of stand-alone standards to an already overcrowded curriculum would be unwelcome to say the least.
The breadth and variety of spatial thinking and of spatial thinkers pose an educational challenge. How can we design instructional programs to fit such a rich, diverse, complex, and intriguing set of learning tasks and domains? Clearly, education in spatial thinking must be strengthened. However, this should not add another layer to an increasingly complex and congested curriculum structure or overburden teachers. Spatial thinking is a missing link across the curriculum and it will help to make the curriculum more coherent. It is an invaluable approach to achieving existing curricular objectives. Spatial thinking is a fundamental process skill that transcends the bounds of particular disciplines. While it is central to the sciences, it is applicable in most, if not all, subject areas. It runs across the curriculum and extends from kindergarten through to twelfth grade (and beyond). It is increasingly possible to support the training of specific skills in spatial thinking and to foster a generation of students who are educated to think spatially. Moreover, because of emerging technologies, spatial thinking is more readily possible, and more challenging skills are being demanded and used because of the rapid evolution and widespread diffusion of technology. Technical systems for support leverage the human capacity for spatial thinking in many ways. They can speed up routine operations, manage massive data sets, generate alternatives, allow easy communication between people, and display results. Therefore, in Chapter 6, the committee develops a position statement about the nature and role of support systems in the K–12 context.