8
Approaches to Fuel Economy and Regulations
This chapter examines the broad variations in medium-and heavy-duty vehicles and explains how the complex nature of trucks influences regulatory options. It explores metrics that capture the work task of the vehicle, thereby providing a means for comparing the relative fuel consumption performance of vehicles on the basis of task. The chapter concludes with a discussion of regulatory approaches and includes examples of fuel consumption regulatory instruments that may be suitable for implementation.
Thirty years ago, the National Highway Traffic Safety Administration (NHTSA) of the U.S. Department of Transportation was faced with the question of how to design a new fuel economy regulation for passenger vehicles. For many important elements, NHTSA was able to build off an existing exhaust emissions program. The regulated parties (vehicle manufacturers), test method (chassis dynamometer), and test cycle (federal test procedure) were adopted by NHTSA for its new fuel economy program. Of course, there were many important elements of the program that were unique to the new fuel economy regulation, such as the method of allowing compliance by means of a corporate average of annual sales and the development of a second test cycle to reflect highway operation.
Today, while there is an existing heavy-duty vehicle exhaust emissions program with its own regulated entities (engine manufacturers), test method (engine dynamometer), and test cycles (Federal Test Procedure [FTP], Supplemental Emissions Test [SET], Ramped Mode Cycle [RMC], and in-use tests), there are factors associated with the U.S. vehicle market that make fuel consumption regulations more difficult and complicated than the design of fuel economy standards for passenger vehicles. Consider the following three examples:
-
The heavy-duty vehicle market is extremely diverse, with a wide range of vehicle types, sizes, and duty cycles.
-
Heavy-duty vehicle manufacturing is driven by customer specifications, which often leads to a far greater variety of pairings between major components (e.g., engine, transmission, chassis, axles, wheels, body shape).
-
Unlike passenger vehicles, vehicle manufacturing is often split between two different manufacturers: the producer of the chassis and a second manufacturer that purchases the chassis, adds a body and special equipment, and ultimately sells the vehicle to the consumer (see Figure 8-1). The exception is pickup trucks and truck tractors, which are completely assembled by the final manufacturer.
PURPOSE AND OBJECTIVES OF A REGULATORY PROGRAM
The purpose and structure of a regulatory program should be as follows: (1) generate cost-effective reductions in fuel consumption from medium- and heavy-duty vehicles, maximizing the savings of fuel at a justifiable cost imposed on the industry and society; (2) accelerate the research, development, and market penetration of new and existing energy saving technologies; (3) reduce the amount of energy consumed per movement of freight or passengers; (4) build on existing market incentives and company practices to lower fuel consumption; and (5) minimize additional administrative burden upon the regulated industry.
There are a handful of major technical and policy questions that must be addressed when developing a new regulatory program. Each is discussed in turn throughout this chapter:
-
Regulated vehicle types. What types of vehicles should be regulated?
-
Regulated parties. Who should the regulated parties be?
-
Metrics for fuel consumption. What metric should be used to measure performance?
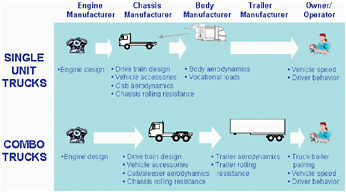
FIGURE 8-1 Shared responsibility for major elements that affect heavy-duty-vehicle fuel efficiency. SOURCE: Bradley and Associates (2009).
-
Methods for certification and compliance. What methods will be used to determine compliance and overall program effectiveness?
-
Regulatory model.
REGULATED VEHICLE TYPES
The committee has considered a broad range of vehicles. These include pickup trucks, transit buses, motor coaches, school buses, delivery vans, straight trucks, and combination vehicles such as tractor trailers. The largest fuel use from the heavy-truck fleet is associated with the vehicles that move the vast majority of the freight: Class 8 tractor trailers with gross combined weight (GCW) ranging from 80,000 lb on the interstate and in excess of 130,000 lb on some state highways (GCW varies considerably, as it is governed by federal and state size and weight regulations). This is not surprising, considering the huge jump in weight hauling capacity between Class 8 (in excess of 130,000 lb) and the rest of the heavy-duty fleet (Class 2b through 7 weight capacity ranges from 8,500 to 33,000 lb). Class 8s are about 20 percent of the fleet in total number of vehicles, but 61 percent of the fuel use of all heavy-duty vehicles. The second largest fuel use segment is the Class 2B, which makes up the majority of heavy-duty vehicles (53 percent) and which is responsible for just under 20 percent of fuel consumption. The third largest class is Class 6. These are considered medium heavy-duty and generally have only a single rear axle, while Class 8 vehicles typically have tandem drive axles. Class 6 vehicles make up about 16 percent of the heavy truck population and consume 11 percent of the fuel. Table 8-1 gives more detail.
Most Class 8 vehicles are combination trucks for which several trailer options are available to complete the vehicle system (see Figure 8-2), adding another dimension to an already complex regulatory challenge. For example, the type of trailer used will influence the vehicle’s overall aerodynamic drag coefficient and the projected frontal area, both of which influence aerodynamic losses and directly affect fuel consumption. The tires, on both the tractor and the trailer, will influence the rolling resistance. In addition, weight and dimension regulations define the “legal” GVW, which also influences fuel consumption. An added complication is that the size and weight regulations for a given vehicle vary depending on the jurisdiction—federal or state.
The problems are compounded further in that vehicles
TABLE 8-1. Mileage and Fuel Consumption by Vehicle Weight Class
Vehicle |
Population (millions) |
Annual Miles (million) |
Annual Fuel Use (million gallons) |
Percent of Population |
Percent of Annual Miles |
Percent of Fuel Use |
Class 2B |
5.800 |
76,700 |
5,500 |
52.8 |
35.1 |
19.3 |
Class 3 |
0.691 |
9,744 |
928 |
6.3 |
4.5 |
3.3 |
Class 4 |
0.291 |
4,493 |
529 |
2.6 |
2.1 |
1.9 |
Class 5 |
0.166 |
1,939 |
245 |
1.5 |
0.9 |
0.9 |
Class 6 |
1.710 |
21,662 |
3,095 |
15.6 |
9.9 |
10.9 |
Class 7 |
0.180 |
5,521 |
863 |
1.6 |
2.5 |
3.0 |
Class 8 |
2.154 |
98,522 |
17,284 |
19.6 |
45.1 |
60.8 |
Total |
10.992 |
218,580 |
28,444 |
100.0 |
100.0 |
100.0 |
SOURCE: DOT (2002). |
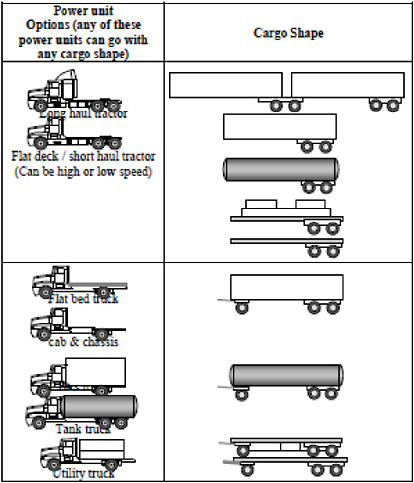
FIGURE 8-2 Illustration of diversity of trailer and power unit (tractor) options.
haul freight of various shapes, sizes, and densities and are often loaded below capacity. While van trailers present a convenient and predictable space envelope for aerodynamic evaluation, other trailers such as flat-decks have no such fixed outer shell, and therefore the shape of flat deck trailers will change with each load transported, which in turn influences aerodynamic drag and thus fuel consumption.
Given the complexity and challenge of establishing a new regulatory policy for medium- and heavy-duty vehicles, there could be a tendency to narrow the scope of a regulation, or at least to focus early implementation, on the largest fuel users in the fleet. This would suggest focusing on Class 8, Class 6, and Class 2b vehicles to cover roughly 90 percent of the fuel use in the medium- and heavy-duty vehicle fleet.
While starting with a subset of heavy-duty vehicles is tempting, there are several drawbacks that should be considered. Uneven policy application will cause disruptions in the marketplace and create the potential for reclassifying various classes of vehicles, as has been done in light-duty vehicles (LDVs), in which cars were reclassified as light trucks to achieve less stringent regulatory standards. Related to “reclassification” is a potential for change in market behavior to avoid higher prices due to regulation (i.e., if Class 2b is regulated but not Class 3 then buyers might buy more of the larger Class 3 trucks because they would become less expensive relative to 2b trucks).
Second, while regulation of medium- and heavy-duty vehicles is complicated, there could be further complications created by seeking to draw artificial lines between various segments. For example, Class 8 straight/vocational trucks typically have two drive axles and Class 7 trucks have one. Class 5 and Class 6 trucks are similar to each other, and Classes 2b and 3 tend to be more similar to each other.
Third, when considering a regulatory framework, there are a number of important parameters beyond fuel use, such as cost effectiveness, equity among manufacturers, potential for gaming, minimizing unintended outcomes, and technology potential. Finally, Congress instructed NHTSA to estab-
lish fuel economy standards for medium- and heavy-duty vehicles—not a portion or subset of medium- and heavy-duty vehicles.
REGULATED PARTIES
There are at least two principal considerations to be evaluated when seeking to determine the most effective point of regulation. The first practical consideration is that the number of regulated entities must be a manageable number (in the tens rather than the hundreds) of parties to limit compliance and administrative burdens. Second, to be effective, the regulation must affect the corporate parties with the greatest control and authority over vehicle design and over those components that offer the potential for substantial reductions in fuel consumption.
Market Concentration
As discussed in Chapter 2, the majority of production of commercial trucks is concentrated in about 12 major corporations that control different portions of the market. Manufacture of class 8 trucks (tractors and straight) is dominated by four companies (Daimler AG, Volvo, PACCAR, and Navistar) that account for more than 90 percent of U.S. truck registrations. The smallest heavy-duty vehicles—Class 2b to Class 4—are dominated by the Big 3 U.S. auto manufacturers with 89 percent of registrations.
Large original equipment manufacturers (OEMs) with significant engineering capability design and manufacture almost all Class 2b, 3, and 8b (semitractors) vehicles. These OEMs have design control over features that determine the completed vehicle aerodynamics. In some cases, these OEMs make the engine and driveline components, while in others these are outsourced to specialist suppliers, who could be given responsibility for regulatory compliance of the power train. For Classes 4 through 8a, there is a mix of vehicles made by large and small OEMs and smaller final-stage manufacturers. The vehicle mix includes box trucks, bucket trucks, school buses, transit buses, motor coaches, refuse haulers, and dump trucks (see Chapter 6). These small entities purchase the chassis, engine, driveline, and in some cases complete cab and chassis units from suppliers who could be made responsible for regulatory compliance. In some cases the supplier content will determine aerodynamic characteristics, but in many cases, the final-stage manufacturer will significantly influence aerodynamic characteristics. Given the very limited engineering resources available to these smaller OEMs, it is unreasonable to assume that such small companies can conduct the necessary aerodynamic tests for each specialty vehicle produced.
Control Over Design and Important Components
A vehicle may be considered as consisting of three major energy-consumption-related components that are separable from one another. These are (1) the wheels and tires yielding tire rolling resistance, (2) the body-yielding aerodynamic losses and (3) the power train. Arguably there is a fourth notable category of energy consumption-auxiliaries, that be considered, but is not as clearly separable. Vehicle chassis represents a structure that simply connects the body and the power train, and may be regarded as part of the power train or part of the body for testing purposes. These are the key features that affect fuel consumption in some way. The vehicle weight and aerodynamics are affected by the hood, cab, skirts, bumpers and overall shape and design of the vehicle body. The power train consists of the engine, hybrid components, transmission, differentials, and drive axles. In considering the entity to regulate, those responsible for the total vehicle or for each/any of these major components are among the options.
Table 8-2 lists the advantages and disadvantages of each choice of regulated party. Regulating at the point of the engine manufacturer is likely to impose the lowest additional administrative burden but limits the program to a small subset of potential reductions in fuel use. A second option is to impose regulation on the power train integrator, which could be an engine company partnered with a drive train component supplier (e.g., hybrid component manufacturers), or a power train integrator could be an integrated truck OEM with selected suppliers. This option would benefit from accounting for engine and power train improvements, but would, in some cases, require greater integration of the existing industry. Regulation of the vehicle manufacturers offers the greatest amount of potential improvements while limiting the number of regulated parties, but the administrative burden will be substantially higher, particularly for smaller vehicle manufacturers.
In the final analysis, the concept of addressing the power train, aerodynamics, and tires seems to have strong potential for success as it would maintain focus on the dominant fuel-consumption-related components of vehicles. The suppliers of these components are arguably in the best position to control future improvements of the components that they manufacture. The final stage manufacturers need some means of assurance that they receive accurate and meaningful data from the suppliers in order to evaluate the final vehicle fuel consumption. Therefore, the point of regulation would need to be at the final-stage vehicle manufacturer, supplemented by the provision of consistent component performance data by the component manufacturers. Annex 8-1 presents a more detailed analysis of a methodology that might underlie a component-based regulatory program.
A perplexing problem for any option, regarding Class 8 vehicles, is what to do about the trailer. The trailer market represents a clear barrier with split incentives, where the owner of the trailer often does not incur fuel costs, and thus has no incentive to improve aerodynamics of the trailer itself or to improve the integration of the trailer with the tractor or truck. Furthermore, legal authority is tenuous, given that trailers are not self-propelled vehicles. One option could be
TABLE 8-2 Advantages and Disadvantages of Each Choice of Regulated Party
Regulated Entity |
Advantages |
Disadvantages |
Engine manufacturer |
|
|
Power train integrator |
|
|
Final stage vehicle manufacturer |
|
|
Fleet owner Vehicle owner |
|
|
to allow manufacturers to certify for additional credits if an improved trailer design and/or integration is satisfactorily incorporated as a complete vehicle. However, in many cases tractors change semitrailers frequently, making integration difficult without standardization of design.
METRICS FOR FUEL CONSUMPTION
Considering the complexity of heavy-duty vehicles and the highly specialized nature of vehicle design and operation with respect to vehicle task, the following advisory principles were developed by the committee:
-
The metrics should incentivize subcomponent and total vehicle development.
-
The metrics should relate to the transport task or vehicle vocation.
-
The metric should encourage energy conservation for a given task.
-
The metric should be based on energy or fuel consumption—e.g. equivalent diesel gallons/cargo tonmile. (See discussion in Chapter 2.) Normalizing to equivalent diesel fuel permits fair comparison across fuel type as energy density varies with fuel type and specification.
The committee recognized that an equipment specification regulation was an option, considering the ongoing SmartWay program as an example. However, a performance-based metric is strongly recommended as it will more adequately address the advisory principals above.
The practical effect of using a gallon per mile metric is that it will result in improvements only to the vehicle itself and in all likelihood encourage smaller vehicles with smaller payloads, resulting in serious erosion of transportation efficiency. On the other hand, the load specific fuel consumption (LSFC) metric such as gallons per cargo tonmile will promote technical improvements and configuration development that increase the amount of cargo that can be carried for a given amount of fuel consumed. Improvements can be achieved in two ways under an LSFC metric: (1) by improving the efficiency of the vehicle (power train, tires, aerodynamics, etc.), the vehicle can move a given amount of freight with lower fuel consumption; (2) by increasing the cargo capacity of the vehicle, the regulated party will also be able to improve its fuel efficiency rating—independent of any change in truck subcomponent fuel efficiency. In combination these two distinct approaches will provide the greatest potential for energy conservation and savings.
Smaller-class single-unit trucks and buses have design and operating characteristics that are different from larger vehicles. For example, utility trucks used by electric power companies may be equipped with a bucket crane and not carry any substantial cargo. Clearly it would not be practical to evaluate the performance of such a vehicle in terms of the mass of transported cargo. Single-unit trucks may also be placed into service towing trailers with a drawbar hitch as shown in Figure 8-2, which is common in the West and
which further complicates assigning an operating weight to the truck. Considering the multiplicity of factors influencing fuel consumption and the complexity of larger vehicle systems and operations, the committee concludes that the notion of a single metric being applied identically to all classes of vehicles appears to be problematic. However, the committee is confident that a standard measurement protocol coupled with different standards and metrics will provide a means of assessing fuel consumption on the basis of work task for medium- and heavy-duty vehicles.
Class 2b and 3 vehicles tend to be higher volume general-purpose vehicles with less custom built content. The high production volume of this vehicle class is conducive to a more general metric such as gallons per mile, gallons per mile per person weight, or gallons per ton mile. Buses also have substantial variability. Of the bus categories, the long-distance motor coach not only transports passengers but they also transports freight, and therefore the task-based metric would need to consider both freight (baggage and package cargo) and passenger mass. Passenger mass can be estimated using “typical passenger” mass multiplied by the number of available seats. Freight mass can be estimated by using a “typical” freight density term multiplied by the cubic capacity of available cargo space.
Implications of Cargo Density for Fuel Consumption Evaluation
For most truck transportation, the nature of the freight task can be classified as volume limited or mass limited. Mass-limited freight is of sufficiently high density that the GVW will be reached before the volumetric capacity of the vehicle is fully utilized. Volume limited freight is of sufficiently low density that it occupies the available cargo space before the GVW is achieved. It is estimated that the split between volume-limited and mass-limited freight on the U.S. highway network is approximately 50/50. Vehicles are often designed on the basis of mass or volumetric capacity, and the characteristics of these vehicles are somewhat sensitive to the methods used to calculate fuel consumption. The following example illustrates practical considerations that will be necessary when developing a fuel consumption regulatory instrument.
Consider the real-world example of two tractor trailers having identical power units but with trailers of different cargo mass capacity and identical volumetric capacity (Figure 8-3). Vehicle A has a GVW of 80,000 lb and a cargo capacity of 48,000 lb. Vehicle B has a GVW of 97,000 lb and a cargo capacity of 61,000 lb (allowing 4,000 lb for the extra axle, suspension and additional trailer structure). Both trailers have identical cargo volume capacity of 3,650 ft3.
It is clear that Vehicle A is better suited to cargo weighing 48,000 lb or less, and vehicle B is better suited to cargo weighing more than 48,000 lb. There is no difference in the volumetric capacity of these vehicles; therefore, the cargo mass dictates the vehicle choice. On the surface this case appears to be ideally suited to the vehicle mass fuel consumption metric (gal/cargo ton-mile). However, when examined more closely, it is apparent that the identical tractor would have different fuel consumption values for each of the two cases given the difference in the GVW and cargo mass capacity. It is likely that if the mass metric were applied, Vehicle B would always outperform Vehicle A (assuming that a proportionate cargo mass is used). This would be counterproductive for low-density, volume-limited freight applications because the mass metric would encourage heavier capacity vehicles with higher tare weight. In such cases, this problem can be offset by considering an alternate metric such as gallons per cargo ft3-mile. Further discussion of alternative metrics can be found in Annex 8-2 to this chapter.
METHODS FOR CERTIFICATION AND COMPLIANCE
The choices of possible methods for certification and compliance of fuel consumption standards for medium and heavy duty vehicles involve some of the most challenging regulatory design issues.
One broad choice pertains to whether it would be possible to establish average standards by corporate entity, as is done under the light duty vehicle CAFE program, or whether the breadth and diversity of the medium and heavy-duty vehicle market precludes such an option. In general there are important benefits associated with a corporate average standard in that it allows corporations flexibility to focus improvements on vehicle types within the retooling cycle. The challenge with a corporate average standard is that the medium- and heavy-duty vehicle market is extremely diverse and would require establishing categories of vehicles by type and application. In addition, the light-duty vehicle CAFE program placed full line, largely domestic, manufacturers at

FIGURE 8-3 Identical tractors used to pull trailers of different mass capacity but identical volume capacity.
a competitive disadvantage due to the form of the regulatory standard. The committee therefore strongly urges NHTSA to proceed with caution if it considers a corporate average standard. Another type of regulatory flexibility is to allow manufacturers to average emissions across engine families. This approach could be used to allow manufacturers to average fuel consumption across vehicle lines. NHTSA would need to perform a separate analysis on the market structure of various truck manufacturers to understand the pros and cons of setting corporate average standards within vehicle types and categories, as well as examining other regulatory flexibilities.
It is clear that the regulatory system should incentivize the subcomponent manufacturers to make real gains in efficiency, but this could be achieved even if the point of regulation is at the OEM. The engagement of a purchaser in seeking highly efficient vehicles is very different from what is typical in the passenger car market. One of the main thrusts of a fuel consumption regulation for medium- and heavy-duty vehicles should be to ensure that the customer has access to reliable data that are based on performance metrics related to the intended function of the vehicle.
Approaches to characterizing or certifying heavy-duty vehicle fuel consumption toward a standard are summarized in Table 8-3. The options range from testing assembled vehicles to modeling and simulating assembled vehicles with most testing at only the power train, tires, and aerodynamics component levels. Any procedure must characterize the consumption or efficiency using a duty cycle that is reasonably representative of real use. The greater the degree of representation, the greater will be the number of test cycles required to cover the applications, but the greater will be the accuracy of the process in reflecting real-world data. A high level of fidelity between regulatory cycles and the real world is required to enable regulators to make the correct decisions and drive the market in the desired direction. Vehicle pur-
TABLE 8-3 Options for Certification of Heavy-Duty Vehicles to a Standard
Method |
Equipment |
Advantages |
Disadvantages |
In-use test (complete vehicle) |
400- to 600-mile test course(s) on public roads |
|
|
Test track (complete vehicle) |
Closed, 1- to 5-mile oval or circular test track |
|
|
Chassis dynamometer |
Heavy-duty chassis dynamometer with data from a coast-down test track |
|
|
Engine test plus vehicle simulation modeling |
Engine dynamometer Vehicle simulation model |
|
|
Power train test plus vehicle simulation modeling |
Engine dynamometer that will accommodate hybrid power train hardware and model/cycle control (CIL) Vehicle simulation model |
|
|
Simulation of entire vehicle |
Vehicle simulation model |
|
|
SOURCE: Modified from Bradley and Associates (2009). |
chasers require an even higher level of fidelity to make the best decisions when specifying a new vehicle. Sophisticated larger fleets will often change engine, transmission, tire, or even OEM selection to gain a 1 or 2 percent fuel consumption reduction. Achieving this level of fidelity is a major challenge.
As discussed previously, using the results from existing engine dynamometer testing for heavy-duty vehicles would allow for accurate, repeatable comparisons, but there are substantial drawbacks to limiting the scope of the rule to only engine technologies. For example, there is a potential lack of fidelity between the dynamometer test cycle and real world performance. Table 8-3 describes the four major widely used test methods—in-use testing, test track testing, chassis dynamometer testing, and simulation modeling—and identifies the advantages and disadvantages of each approach. A final method, generally used in power train development to test the combined engine and drive train, would require the engine dynamometer test cycle to utilize the load characteristics of real trucks over real duty cycles. The load on the engine would be determined by a vehicle simulation, an approach that bears some similarity to the approach used in Japanese regulations. The truck and trailer simulation (aerodynamics, tires, mass) could include applicable fuel-saving features to represent a range of truck models, unlike the fixed truck characteristics defined in Japan’s model. To carry this concept to hybrid vehicles, the “engine” would need to be augmented with the hybrid components and thus become a “power train” in the test cell (also called component in the loop; CIL). As the interaction between engines and conventional (nonhybrid) transmissions becomes increasingly emphasized, the concept of evaluating the performance of a complete power train retains merit.
Three of the methods listed in Table 8-3 for determining vehicle fuel consumption require the use of complete vehicles: in-use testing, test track testing, and chassis dynamometer testing. Vehicles that operate with trailers would need to have standard trailers for the testing. In the case of Class 8b vehicles, at least two trailer types would be required: a standard box van trailer to be tested on tractors intended for this type of application, and a low frontal area trailer for trucks to be used with other trailer types.
Two of the methods for determining vehicle fuel consumption that are listed in Table 8-3 require only component-level testing. These are the engine test plus vehicle simulation approach, and the power train test plus vehicle simulation. The final method listed in Table 8-3 is pure simulation, although even this approach will require some testing to validate the data used in the simulation model.
Sample Applications of Methods to Vehicle Classes
For Class 2b vehicles and Class 3 pickup trucks, a chassis dynamometer test for fuel consumption similar to the test used in light-duty vehicles is a viable option. These vehicles are often used in ways similar to light duty vehicles, and existing test facilities could be used. Many of these vehicles are also made in relatively high volume, making a full-vehicle test less difficult to manage for the manufacturer. An ability to rely on existing industry and regulator experience and capability makes this approach attractive.
In medium- and heavy-duty vehicles from Class 3 up through Class 8a, manufacturing volumes are often low and many different configurations are built on a given platform. This makes chassis dynamometer fuel consumption testing much more difficult and expensive than in smaller vehicles. For these larger vehicles it makes sense to combine engine or power train test data with vehicle simulation models. Particularly in the case of hybrid vehicles, it will be important to have high-fidelity data for the fuel consumption and performance of the power train. This data may come from testing, simulation, or a blend of simulation such as hardware-in-the-loop (HIL) or CIL. Even if a pure simulation approach is used, some level of test data is required to validate the models. A wide range of vehicle duty cycles may need to be simulated or tested in order to achieve adequate fidelity with real-world fuel consumption data.
Tractor trailers (Class 8b) are also available in dozens of configurations, many of which are produced in low volume. Once again, chassis dynamometer fuel consumption testing would be difficult and expensive, and so some level of vehicle simulation modeling is likely to be required. Engine or power train test data can be provided as needed. Again, many vehicle duty cycles may need to be simulated or tested in order to achieve adequate fidelity. Regulators will want to reinforce rather than impede the fuel consumption sensitivity in Class 8b, where purchasing decisions are often based on differences as small as 1 or 2 percent in fuel consumption. For example, a buyer will want to know which of 10 or more available aerodynamic treatments will perform best in the buyer’s particular application. Defining tests or simulations that can provide an accurate answer to a question like this will not be easy. Defining a regulatory process and standards that do not drive incorrect decisions by vehicle manufacturers will require considerable care.
For the first iteration of the new regulatory program for medium- and heavy-duty vehicles, the committee recommends that regulators consider test methods that minimize the administrative burden on those vocational vehicles that are not the large fuel users (that is, all vehicles not included in Class 2b, Class 6, or Class 8b line-haul tractor trailers). For these numerous types of vehicles that account for less than 10 percent of commercial truck fuel consumption, the committee recommends two options: (1) pure vehicle simulation, and (2) engine-in-the-loop, also called CIL (engine connected to a dynamometer that emulates the rest of the vehicle). The pure simulation modeling approach allows the regulated entity to piggyback off of existing engine tests and data for other components (e.g., transmission, tires, electric machine). The CIL approach takes advantage of the exist-
ing engine test procedure while also incorporating existing component data. At this point, it appears that pure simulation would be the less expensive option.
When simulation models are used, inputs are required to represent components. Some of these inputs may come from standardized tests, such as a test for tire rolling resistance or a wind tunnel test for aerodynamic drag. These inputs may also come from simulation models, if the models are validated and sufficiently accurate. For example, a CFD model may be used to determine the Cd of a vehicle in place of wind tunnel testing. Data used in a model for regulatory approaches will need to come from a standard test or analysis process that is recognized by the entire industry. Many new test and analysis procedure standards will be needed. It may be necessary to approve both codes and experimental facilities to insure quality control and uniformity in the determination of wind drag.
Figure 8-4 shows an outline of how a power train test can be combined with a vehicle simulation model to determine the fuel consumption of a vehicle. This is the CIL approach. This figure shows a hybrid electric power train with a diesel engine including exhaust aftertreatment. The power train is tested in a test cell, where the dynamometer load is determined by a vehicle model. The vehicle model, in turn, uses input data for parameters such as rolling resistance, mass, and aerodynamic drag. The vehicle model is exercised over a specified route, and the resulting power demands are applied on the power train by the dynamometer. The resulting fuel consumption is experimentally measured.
The choice of test cycle is a critical part of any vehicle fuel consumption test or simulation. Test cycles selected for regulatory use will need to reflect real-world duty cycles to the extent possible. Parameters of importance include maximum speed, average speed, speed fluctuation, number of stops, and amount of idling. It will not be possible to faithfully reproduce the duty cycle to be experienced by every vehicle, so similar applications will be represented by one or a few duty cycles for regulatory purposes.
Overall Regulatory Structure
Introduction
Applying a regulatory system that pushes technology in the drive train, tires, and vehicle shape (aerodynamics) ensures that incentives are applied at the foundation of the major vehicle systems that influence fuel consumption.
Given the high fuel consumption sensitivity of some medium- and heavy-duty vehicle purchasers, it appears that one priority should be to ensure that accurate information on the fuel consumption characteristics of a completed vehicle is available to the purchaser. Having such information would help drive the selection of vehicles with the lowest fuel consumption for the task performed. The notion of regulating the final-stage manufacturer and including a requirement on the component manufacturers to provide relevant performance data to the purchaser will be an important part of the regulation.
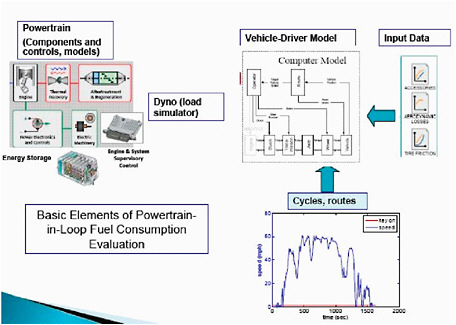
FIGURE 8-4 CIL test of a hybrid vehicle power train to determine vehicle fuel consumption on a specific test route.
Focusing on the power train, aerodynamics, and tires provides a means of incentivizing these three important areas. Measuring and documenting the performance of these key components in a constant and transparent way will be important for the final-stage manufacturer. NHTSA may wish to require suppliers to provide information in standardized form at the power train, aerodynamics and tire levels. The final-stage manufacturer would have the responsibly of combining the performance of these components to achieve the lowest cost for the intended vehicle task while complying with the regulatory fuel consumption requirement. The task-based fuel consumption metrics would be used by the final stage manufacturer to inform the customer as part of a labeling requirement.
In summary, the concept of this example regulatory model is as follows:
-
Major components such as power train, tires, and aerodynamics (including factors for accessories and auxiliaries) would each be tested or simulated, and efficiency data made available to OEMs in a common format using industry standard metrics and procedures.
-
The completed vehicle would be regulated, and in addition customer specific data would be provided that would inform the vehicle purchaser about the fuel consumption performance of the particular vehicle in relation to the intended task. This form of regulation would simplify the regulatory task compared to other alternatives and provide the flexibility needed to address the complex nature of the industry.
Compliance, Audit, Enforcement
Chapter 3 describes the compliance audit process for heavy-duty engines and for passenger car emissions. The committee believes that similar methods would be adequate to audit compliance for heavy-duty vehicle fuel consumption. The use of models and simulation in the certification process could cause a complication in auditing in that the audit test on a real vehicle might need to be assessed against a model output generated for certification. This concern would need to be addressed in the design of the regulation.
Pilot Program
The committee recommends that NHTSA conduct a pilot program to “test drive” the certification process and validate the regulatory instrument proof of concept. There are two broad purposes for such a pilot program. In the first element, the agency would gain experience with certification testing, data gathering, compiling, and reporting. There needs to be a concerted effort to determine the accuracy and repeatability of all test methods and simulation strategies that will be used with any proposed regulatory standards and a willingness to fix issues that are found. There are numerous technical challenges related to implementation of this program (e.g., reliable and accurate methods to determine tire rolling resistance and vehicle aerodynamic drag coefficients, incorporation of simulation modeling with hardware, integrating a hybrid drive train within the standard test cell, characterizing subcomponents for use in simulation modeling). This trial period will serve as a means for developing and refining the regulatory processes before the official start date of the program.
A second element would include gathering data on fuel economy from several representative fleets of commercial trucks (e.g., long-haul, delivery vans, specialty vehicles, and large pickups). These data would continue to be collected once the program was established in order to provide a real world check on the effectiveness of the regulatory design on the fuel economy of trucking fleets in various parts of the marketplace and in various regions of the country. As this program will place an additional administrative burden on NHTSA and private operators, the committee recommends that Congress consider an annual funding allocation for this program.
Concluding Comments
This is an important juncture. The choices that will be made over the course of the next few years will establish the regulatory design for medium- and heavy-duty-vehicle fuel consumption standards for the next several decades at least. Although the stringency of the standards themselves may be revisited from time to time, the regulatory design elements (regulated parties, certification tests and procedures, compliance methods)—once established—are far more difficult to modify.
In many cases the commercial vehicle market is sophisticated, driven by knowledgeable purchasers who focus on the efficiency of their operations, including the fuel costs associated with accomplishing their tasks. Thus, one of the most important challenges facing NHTSA is how to enhance and improve upon the commercial truck industry’s existing incentive to maximize fuel economy of its trucks and fleets.
At the same time, there are commonly acknowledged characteristics in the commercial marketplace for trucks and buses that may be improved by a regulatory approach, such as split incentives between owners and operators (e.g., trailers), and the short payback period of 18 months to 2 years, that create barriers to the adoption of efficiency technologies for many purchasers. The existence of technology packages for some vehicle classes that offer significant fuel consumption reduction potential at reasonable costs suggests that well-designed policies to overcome problems such as split incentives or too short a payback period may yield important benefits (see Table 6-19).
Due to the complexity of the vehicle market the commit-
tee was not able to give adequate consideration to the non-commercial markets such as personal pickup trucks, school buses, personal motor homes. NHTSA should consider these applications in their regulatory proposal.
A fundamental concern raised by the committee and those who testified during our public sessions was the tension between the need to set a uniform test cycle for regulatory purposes, and existing industry practices of seeking to minimize the fuel consumption of medium and heavy-duty vehicles designed for specific routes that may include grades, loads, work tasks or speeds inconsistent with the regulatory test cycle. This highlights the critical importance of achieving fidelity between certification values and real-world results to avoid decisions that hurt rather than help real-world fuel consumption.
Because regulations can lead to unintended consequences, either because the variability of tasks within a vehicle class is not adequately dealt with or because regulations may lead to distortions between classes in the costs of accomplishing similar tasks, the committee urges NHTSA to carefully consider all factors when developing its regulatory proposal.
FINDINGS AND RECOMMENDATIONS
Regulated Vehicle Types
Finding 8-1. While it may seem expedient to focus initially on those classes of vehicles with the largest fuel consumption (i.e., Class 8, Class 6, and Class 2b, which together account for approximately 90 percent of fuel consumption of medium- and heavy-duty vehicles), the committee believes that selectively regulating only certain vehicle classes would lead to very serious unintended consequences and would compromise the intent of the regulation. Within vehicle classes, there may be certain subclasses of vehicles (e.g., fire trucks) that could be exempt from the regulation without creating market distortions.
Regulated Parties
Finding 8-2. Large OEMs, which have significant engineering capability, design and manufacture almost all Class 2b, 3, and 8b vehicles. Small companies with limited engineering resources make a significant percentage of vehicles in Classes 4 through 8a, although in many cases they buy the complete chassis from larger OEMs. Regulators will need to take the limitations of these smaller companies into account.
Finding 8-3. Commercial trailers are produced by a separate group of about 12 major manufacturers that are not associated with truck manufacturers. Trailers, which present an important opportunity for fuel consumption reduction, can benefit from improvements in aerodynamics and tires (see Chapter 5 for details).
Recommendation 8-1. When NHTSA regulates, it should regulate the final-stage vehicle manufacturers since they have the greatest control over the design of the vehicle and its major subsystems that affect fuel consumption. Component manufacturers will have to provide consistent component performance data. As the components are generally tested at this time, there is a need for a standardized test protocol and safeguards for the confidentiality of the data and information. It may be necessary for the vehicle manufacturers to provide the same level of data to the tier suppliers of the engines, transmissions, and after-treatment and hybrid systems.
Recommendation 8-2. Separate regulation of trailer manufacturers will be necessary to promote more fuel-efficient trailers, including integration of the trailer design with the tractor for improved aerodynamic performance, lower tare weight, and a requirement for low-rolling-resistance tires.
Fuel Consumption Performance Metrics
Finding 8-4. Since the primary social benefit of the medium- and heavy-vehicle sector is the efficient and reliable movement of freight, movement of purpose-built integrated equipment, or performance of a task, it is necessary to establish a metric that includes a factor for the work performed (e.g., gallons per cargo ton-mile rather than simply gallons per mile) to ensure that the regulatory instrument meets societal goals.
Finding 8-5. Choosing a metric associated with the movement of freight will promote improvements that increase the amount of cargo that can be carried per unit of fuel consumed, and thus provide a means of quantifying the benefits of more productive vehicles that move the same amount of freight with fewer trips and fewer vehicle-miles traveled, such as longer combination vehicles (LCVs).
Finding 8-6. Setting a metric based exclusively on gallons per cargo ton-mile (gal/ton-mile) may not adequately address light-density freight that is limited by volume.
Recommendation 8-3. NHTSA should establish fuel consumption metrics tied to the task associated with a particular type of medium- or heavy-duty vehicle and set targets based on potential improvements in vehicle efficiency and vehicle or trailer changes to increase cargo-carrying capacity. NHTSA should determine whether a system of standards for full but lightly loaded (cubed-out) vehicles can be developed using only the LSFC metric or whether these vehicles need a different metric to properly measure fuel efficiency without compromising the design of the vehicles.
Methods for Certification and Compliance
Finding 8-7. The regulatory certification and compliance options discussed in this report are the product of much discussion and thought by committee members, supported by input from industry, government, and other organizations. Some certification and compliance methods seem more practical than others, and the committee acknowledges that there may be other options or variations that have yet to be identified. Nevertheless, the committee has determined that regulating the total vehicle fuel consumption of medium-and heavy-duty vehicles will be a formidable task due to the complexity of the fleet, the various work tasks performed, and the variations in fuel-consumption-related technologies within given classes, including vehicles of the same model and manufacturer.
Finding 8-8. A certification test method must be highly accurate, repeatable, and identical to the in-use compliance tests as is the case with current regulation of light-duty vehicles tested on a chassis dynamometer, and for heavy-duty engine emission standards tested on engine dynamometers.
Finding 8-9. Using the process and results from existing engine dynamometer testing for criteria emissions to certify fuel economy standards for medium- and heavy-duty vehicles would build on proven, accurate, and repeatable methods and put less additional administrative burden on the industry. However, to account for the fuel consumption benefits of hybrid power trains and transmission technology, the present engine-only tests for emissions certification will need to be augmented with other power train components added to the engine test cell, either as real hardware or as simulated components. Similarly, the vehicle attributes (aerodynamics, tires, mass) will need to be accounted for, one approach being to use vehicle-specific prescribed loads (via models) in the test cycle. This will require close cooperation among component manufacturers and vehicle manufacturers.
Finding 8-10. At present there is no established federal test method for heavy-duty vehicle fuel consumption. Empirical testing (from components in an emulated vehicle environment to the whole vehicle), simulation modeling, or both may be used for the characterization and certification of regulated equipment. Each approach involves uncertainties that can affect certification and compliance. This finding underscores the need for a pilot regulation program.
Finding 8-11. Significant segments of the medium- and heavy-duty-vehicle purchasing process are highly consumer driven, with many engine, transmission, and drive axle choice combinations resulting in a wide array of completed vehicles for a given vehicle model. From a regulatory standpoint, the use of expensive and time-consuming chassis testing on each distinct vehicle variation is impractical. However, by knowing the effects of the performance of major subcomponents on fuel consumption, it may be practical to demonstrate compliance certification with vehicle standards by aggregating the subcomponents into a specified virtual vehicle for computers to evaluate fuel consumption of the completed vehicle.
Finding 8-12. Further research will be required to underpin the protocol used to measure key input parameters, such as tire rolling resistance and aerodynamic drag forces and to ensure the robustness of simulations for evaluating vehicle fuel consumption. These major components may be assembled through simulation to represent a whole-vehicle system, and models benchmarked to reliable data may be used to extend the prediction to a variety of vehicle types, by changing bodies (aerodynamic measures), tires, and operating weights associated with the power trains.
Recommendation 8-4. Simulation modeling should be used with component test data and additional tested inputs from power train tests, which could lower the cost and administrative burden yet achieve the needed accuracy of results. This is similar to the approach taken in Japan, but with the important clarification that the program would represent all of the parameters of the vehicle (power train, aerodynamics and tires) and relate fuel consumption to the vehicle task. Further, the combined vehicle simulation/component testing approach should be supplemented with tests of complete vehicles for audit purposes.
Finding 8-13. There is an immediate need to take the findings and recommendations in this report and begin the development of a regulatory approach. Significant engineering work is needed to produce an approach that results in fuel efficiency standards that are cost-effective and that accurately represent the effects of fuel-consumption-reducing technologies. The regulations should fit into the engineering and development cycle of the industry and provide meaningful data to vehicle purchasers.
Recommendation 8-5. Congress should appropriate money for and NHTSA should implement as soon as possible a major engineering contract that would analyze several actual vehicles covering several applications and develop an approach to component testing and related data collection in conjunction with vehicle simulation modeling to arrive at LSFC data for these vehicles. The actual vehicles should also be tested by appropriate full-scale test procedures to confirm the actual LSFC values and the reductions measured with fuel consumption reduction technologies in order to validate the evaluation method.
Recommendation 8-6. NHTSA should conduct a pilot program to “test drive” the certification process and validate
the regulatory instrument proof of concept. It should have the following elements.
-
Gain experience with certification testing, data gathering, compiling, and reporting. There needs to be a concerted effort to determine the accuracy and repeatability of all the test methods and simulation strategies that will be used with any proposed regulatory standards and a willingness to fix issues that are found.
-
Gather data on fuel consumption from several representative fleets of vehicles. This should continue to provide a real-world check on the effectiveness of the regulatory design on the fuel consumption of trucking fleets in various parts of the marketplace and various regions of the country.
BIBLIOGRAPHY
Bradley, M.J. and Associates LLC. 2009. Setting the Stage for Regulation of Heavy-Duty Vehicle Fuel Economy and GHG Emissions: Issues and Opportunities. Washington D.C.: International Council on Clean Transportation. February.
Davis, S., S. Diegel, and R.G. Boundy. 2009. Transportation Energy Data Book, Edition 28. Rept. No. ORNL-6984. Knoxville, Tenn.: U.S. Department of Energy, Energy Efficiency and Renewable Energy.
DOC (U.S Department of Commerce), Census Bureau. 2005. 2002 Economic Census, Industry Product Analysis. March. Washington, D.C.
DOE, EIA (U.S. Department of Energy, Energy Information Administration). 2009a. Annual Energy Review 2008. DOE/EIA-0384(2008). Washington, D.C.: EIA.
DOE, EIA. 2009b. Annual Energy Outlook 2009. DOE/EIA-DOE/EIA-0383(2009). March. Washington, D.C.: EIA.
Federal Highway Administration, Highway Statistics Summary to 1995, Table VM-201A and Highway Statistics (annual releases), Table VM-1. Washington D.C.
NESCCAF, ICCT, SwRI. 2009. Regulation of Heavy-Duty Vehicle Fuel Economy and GHG Emissions: Issues and Opportunities. October. Available at www.nescaum.org/.../heavy-duty-truck-ghg_report_final-200910.pdf.
TIAX, LLC. 2009. Assessment of Fuel Economy Technologies for Medium- and Heavy- Duty Vehicles. Final Report. Report to the National Academy of Sciences. Cambridge, Mass. September.
ANNEX 8-1:
COMPONENT-BASED FUEL CONSUMPTION ASSESSMENT METHOD
Introduction
The objective of this annex is to present, in broad terms, an example of a credible methodology for characterizing the fuel consumption of a medium- or heavy-duty vehicle by tests and/or simulation of the major components. This concept considers a certification and labeling approach for the main fuel consumption components that would be aggregated to provide fuel consumption performance of the completed vehicle. This approach recognizes that many fuel consumption improvements occur at the sub component level and provides a means of quantifying the performance of these components so that the final stage manufacturer will have reliable performance data to determine vehicle fuel consumption.
For advanced heavy-duty vehicle designs, the physical power train is not readily classed into separately operating sub-components such as engine and transmission, because there can be a high level of communication between these sub-components and because they are mutually controlled to achieve their function. For example, an engine and a hybrid drive train are inseparable in operation because the control system commands both major subcomponents to achieve propulsion. Even with the lowest technology option of a manual transmission attached via a clutch to an engine, the average human driver determines the engine operating envelope based on the duty cycle, the engine performance map, the transmission ratios and the drive axle ratio. The realistic engine operating envelope is not defined uniquely without these sub-components attached and in use. With some qualification, present day engine testing may not necessarily reflect how the engine will be used in a specific application insofar as the torques and speeds of the engine in use, and the nature of transients in use may not reflect the torques, speeds and transients employed in the test cell.
If simulation and physical testing are equally verifiable as facsimiles of the real-world operation of a vehicle, its components or its sub-components, then simulation and physical testing should be equally valid techniques for use in certification. For example, aerodynamic drag on a body may be found either by wind-tunnel testing or by computer-aided aerodynamic modeling, provided both methods can be shown to be accurate and repeatable. As a further example, the performance characterization of an engine and intelligent transmission combination may be found using a dynamometer in a test cell, or by fully characterizing the engine and transmission separately and combining them with a model for their controller through a simulation exercise. On the one hand, when simulation is used, each component in the simulation must be fully characterized, usually necessitating a larger number of measurements of subcomponents. On the other hand, a simulation proves economical in modeling the effect changes in controls or architecture, or in examining different trucks employing some similar components, since these changes would otherwise necessitate a large number of tests of a whole component or major component subsystem.
The accepted methods used to measure rolling resistance of wheels (with tires), the efficiency of a power train, and the drag characteristics of a body all differ substantially. The existing test methods may need further development to achieve fidelity with real world vehicle operating results.
-
Tire rolling resistance is determined independently and physically in accordance with accepted test protocol. Power train performance is determined physically either by attaching a power train to a dynamometer or by operating a chassis or mule containing a power train with wheels on the drive axle on a chassis dynamometer: in the latter case the wheels and dynamometer rollers are merely connection components between the power train and a rotating dynamometer. Aerodynamic body performance is determined in a wind tunnel. These three test approaches are substantially different and independent from one another, and employ different apparatus.
-
An admixture of these characterizations may be obtained through the coast down of a whole vehicle on the road, but the metrics required are intertwined and the accuracy of the process is challenged by surface and atmospheric effects.
-
Tire rolling resistance may be simulated through computer-aided finite element design models relying on fundamental materials stress and deformation equations. Power train performance may be simulated by using models currently available for whole vehicle simulation, by assembling accurate sub-models of sub-components with links that rely on basic physics (torques and speeds) or precisely defined control algorithms. Aerodynamic body performance may determined using finite element fluid flow models which may vary in their level of empirical tuning. These three modeling processes are substantially different from one another, and employ different types of code for their execution.
The Component-Based Procedure
The terms “measured” and “measurement” below are intended to reflect either output from testing activities or output from modeling or simulation activities, with no preference for either, but with the assertion that either approach requires verified fidelity with regard to accuracy and precision.
The three components, namely body, power train and wheels (with tires), of a vehicle proposed for sale should be measured separately. Certain accessories may also require
independent measurement if they are not easily included in the other three.
Wheels (Including Tires)
The wheels of a vehicle are usually characterized with respect to rolling resistance. The rolling resistance, as a coefficient, represents the horizontal force which must be applied to overcome the internal energy losses of the tire when it supports a given vertical weight on a horizontal surface. The coefficient of rolling resistance (Crr) is reasonably constant with respect to speed and vertical load. The rolling resistance is influenced largely by the tire material construction, wear, and to a lesser degree the road surface.
Aerodynamics
The aerodynamics of the vehicle should be determined by installing a body on a suitable facsimile of wheels and chassis in a wind tunnel, or else by installing a whole vehicle in a wind tunnel. A scaled model may be used where it can be demonstrated by similarity analysis that the resulting measurements may be applied to a full scale measurement. The measurement of interest is the drag force on the body as a function of wind speed over the body. Drag force varies in close proportion to the square of the air speed, and is influenced by air density and by yaw (which results in real vehicle operation from the presence of wind which is not in the direction of travel and which is not very low in speed with respect to the vehicle speed.) Computer simulation of air flow over the body may be used to infer the drag force, provided appropriate controls are used.
Certain trucks and tractors simply would not benefit from some aerodynamic accouterments, either because they are not intended to be driven at high speed, or because they do not carry a box body or tow a box trailer with a large frontal area. For vehicles which are not intended to be driven at high speed, determination of CdA would be purposeless.
Power Train
The power train of the vehicle consists typically of an engine, a transmission, which may include hybrid hydraulic or hybrid electric components, one or more drive axles, possibly an energy storage system, and a control system to manage the components in response to driver commands under constraint of road load. The exhaust aftertreatment system, often considered part of the engine, may evolve to the status of a separate component in future versions of testing.
A power train may be used with a variety of tires and bodies in real vehicle applications, and may be configured or optimized differently for each application, or configured generically for use in several applications.
Vehicle
Different vehicle types, categorized by weight class and use, may each be associated with one or more drive cycles, reasonably corresponding to real use of those vehicles. For example, an over-the-road tractor may be associated with a high-speed cycle, indicative of freeway behavior, and a low-speed cycle, indicative of transient behavior in an urban environment. Consider that the efficiency of a power train is to be measured or modeled, and that its associated application, body and wheels are all defined. These drive cycles exemplifying the application can be translated to a set of hub speed (versus time) and hub torque (versus time) target values, provided that the power train is considered to be propelling this well-defined vehicle. Data which are required to formulate a power train test (physical or simulated) are largely the same as those required to execute a light-duty vehicle chassis test and are as follows:
-
Vehicle mass
-
Tire rolling diameter
-
Effective Crr value for the wheels
-
Effective CdA value or the aerodynamic drag
-
Value for air density
-
Test cycle, as a set of speed versus time values
The power train should then be exercised through the speed-torque target values either physically in a test cell or through simulation. A human driver will be needed, although physical testing and simulation may otherwise employ a driving algorithm, provided that the algorithm reasonably represents a human driver. The choice of driver or driving algorithm must be addressed carefully, because it may impact engine transient behavior and manual transmission behavior substantially. For rapid decelerations, it will be necessary to use friction brakes or a retarder to provide deceleration torque, and for hybrid vehicles the decelerations may be used for energy capture. A physical power train test may also be accomplished with a mule or complete vehicle on a chassis dynamometer, but the dynamometer coefficients measured or projected at the drive hubs must be set to reflect the values of Crr and CdA required for the designated power train test, and not necessarily for the vehicle on which the test is being performed.
Assembled Components
The tires, aerodynamics and power train might all be separately regulated. However, they might also be combined to mimic a completed vehicle. Experimentally, a power train test will provide whole vehicle fuel consumption data if the power train test uses values for aerodynamic drag and for tire rolling resistance which represent that vehicle. However, it is inappropriate to expect that each variant of a vehicle should require a separate power train test. If modeling is used in a
verifiable fashion to mimic the power train and provide efficiency data for a specific vehicle, then the same model may be modified with reasonable confidence to accommodate varying values for aerodynamic drag, rolling resistance, and vehicle weight. In this way, a wide variety of vehicles which use the same power train may be simulated economically.
For any particular power train configuration, the power train performance may be confirmed on two or three cycles using weight, aerodynamic drag and tire rolling resistance suited to a reasonable vehicle type. Data of this kind may be extended through modeling to reveal the power train performance for any test cycle. If several cycles are executed, the variation of fuel consumed with respect to average speed can be computed, and these data would be available for consumer information and as regulatory metrics. As feedback controls become common, power trains will use many sensor inputs to ride up against NTE (Not to Exceed) limits for NOx. This means that actual fuel consumption may vary significantly as a function of ambient temperature, humidity, intake manifold temperature, coolant and oil temperatures, barometric pressure, aftertreatment temperature, aftertreatment aging, and other factors. Some reasonable consensus on standard test conditions will be important for reporting against a fuel consumption standard.
ANNEX 8-2:
ALTERNATIVE METRICS
The measures listed in Table 8-3 address the vehicle task and are based on fuel or energy consumption. This list is not complete as there are many vehicle tasks not covered. This annex elaborates on some of these tasks.
One alternative approach to regulating tractor trailer fuel consumption would be to simply regulate the tractor based on standard loads tied to engine power rating. Tractors could be grouped into power ranges such as low-, medium-, and high-horsepower categories, and corresponding GVW values applied. Each of these power categories would have separate fuel consumption targets. See Figure 8-2-1.
Consider the single vehicle units shown in Figure 8-2-2 with the same GVW rating and the identical power train and chassis. For the purpose of this discussion it is assumed that the flat bed unit is used for heavy loads such as steel or lumber and the box truck is used for lighter density cargo such as courier packages. If the mass metric (gal/cargo ton-mile) were used then there would be strong incentive to minimize vehicle tare weight so that the cargo mass term in the metric could be increased thereby improving performance. This would have a clear benefit for the flat bed truck as the cargo is of sufficient density to benefit from the lighter vehicle. However for the box truck with low density cargo, the incentive for reduced tare weight may not provide any direct benefit to the shipper. In addition, when the mass metric is applied to the box truck, it would give preference to a lighter smaller box which would undermine the volumetric value of the vehicle. A metric based on volume (gal/cargo ft3-mile) would resolve this particular application.